1. Chemical context
Antipyrine (also known as phenazone) derivatives display antioxidant (Bashkatova et al., 2005
), anti-putrefactive (Abd El Rehim et al., 2001
) and optical (Collado et al., 2000
) properties. Among pyrazole analogues, 4-amino-1,5-dimethyl-2-phenylpyrazole-3-one, known as 4-aminoantipyrine, possesses a free amino group. It has received attention because it exhibits various biological activities, such as antifungal, antibacterial, antimalarial, antiviral, anti-inflammatory and antipyretic properties (Nibila et al., 2020
). 4-Aminoantipyrine derivatives are also considered to be model compounds in the biological and medical fields (Senthilkumar et al., 2016
). Schiff bases of 4-aminoantipyrine and their complexes have a wide range of applications in medicinal, analytical and pharmacological areas (Oudar, 1977
; Zyss, 1979
), and they also possess chemotherapeutic properties (Raman et al., 2007
; Alam & Lee, 2016
). As part of our studies in this area, we now report the syntheses and structures of the title compounds, C22H26N4O (I) and C18H16FN3O (II).
A search of the Cambridge Structural Database (CSD, Version 5.43, last update November 2022; Groom et al., 2016
) gave 31 hits for 4-aminoantipyrine structures with a p-substituted benzylidene ring. Of particular interest are the 4-(dimethylamino)benzylidene analogue (CSD refcode TAYLUB01; Asiri et al., 2010
) of I (both compounds crystallize in the monoclinic space group C2/c) and the 4-(chloroamino)benzylidene (KELZIL; Sun et al., 2006
) and 4-(bromoamino)benzylidene (KEQXOU; Yan et al., 2006
) analogues of II (all three compounds crystallize in the orthorhombic space group Pbca). Their molecular structures and Hirshfeld surface analyses are compared to those of the title compounds.
2. Structural commentary
The molecular structures of I and II are illustrated in Figs. 1
and 2
, respectively. Selected geometric parameters for I and II and their analogues are given in Table 1
. The various dihedral angles in the five compounds are given in Table 2
. The configuration about the N3=C12 bond is E, which favours the presence of an intramolecular C12—H12⋯O1 hydrogen bond in both compounds (Tables 3
and 4
, respectively), and in their analogues. The N3=C12 bond length is 1.291 (2) Å in I and 1.289 (2) Å in II. The pyrazole ring mean plane (A = N1/N2/C1–C3; r.m.s. deviations are 0.055 and 0.057 Å for I and II, respectively) is twisted on the N1—N2 bond in both compounds. The phenyl ring (B = C4–C9) and the substituted benzylidene ring (C = C13–C18) are inclined to the pyrazole ring mean plane A by 54.87 (7) and 22.92 (7)°, respectively, in I and by 60.44 (8) and 12.70 (9)°, respectively, in II. The latter two rings, B and C, are inclined to each other by 73.98 (6) in I and by 71.28 (8)° in II. The difference in the conformation of the two structures is illustrated in Fig. 3
showing the structural overlap (Mercury; Macrae et al., 2020
) of molecules I and II. It can be seen from Table 2
that the conformation of I is similar to that of the 4-(dimethylamino)benzylidene analogue (TAYLUB01). However, this is not the case for compound II: while the conformation of the 4-(chloroamino)benzylidene (KELZIL) and 4-(bromoamino)benzylidene (KEQXOU) analogues of II are similar there is a significant difference compared to the conformation of compound II. For example, the A to B dihedral angle is 60.44 (8)° in II but is 51.6 (1) and 50.8 (2)°, in the respective analogues. The other dihedral angles are also significantly different, as seen in Table 2
.
| I | TAYLUB01a | II | KELZILb | KEQXOUc | N3—C12 | 1.291 (2) | 1.288 (2) | 1.289 (2) | 1.276 (2) | 1.279 (5) | C2—N3—C12—C13 | –177.11 (11) | 173.20 (11) | –175.43 (14) | –176.68 (15) | 177.5 (4) | | | | | | | C1—N1—N2 | 109.58 (9) | 109.58 (10) | 108.75 (12) | 108.58 (13) | 106.9 (3) | C1—N1—C4 | 121.78 (10) | 122.30 (10) | 120.50 (13) | 122.40 (13) | 122.4 (3) | N2—N1—C4 | 119.11 (9) | 118.13 (10) | 118.90 (13) | 119.12 (14) | 119.8 (3) | Sum | 350.47 (9) | 350.0 (1) | 348.15 (13) | 350.10 (13) | 349.1 (3) | | | | | | | C3—N2—N1 | 106.23 (9) | 106.50 (10) | 107.40 (13) | 107.34 (13) | 107.7 (3) | C3—N2—C10 | 121.11 (10) | 122.30 (11) | 125.50 (14) | 124.77 (14) | 125.1 (3) | N1—N2—C10 | 114.20 (10) | 114.68 (10) | 118.09 (13) | 117.05 (15) | 115.9 (3) | Sum | 341.54 (10) | 343.48 (10) | 350.99 (13) | 349.16 (14) | 348.7 (3) | Notes: (a) Asiri et al. (2010 ); (b) Sun et al. 2006 ); (c) Yan et al. 2006 ). | |
Dihedral angle | I | TAYLUB01a | II | KELZILb | KEQXOUc | Planes A to B | 54.87 (7) | 55.01 (7) | 60.44 (8) | 51.6 (1) | 50.8 (2) | Planes A to C | 22.92 (7) | 19.03 (7) | 12.70 (9) | 8.7 (1) | 9.1 (2) | Planes B to C | 73.98 (6) | 73.98 (6) | 71.28 (8) | 59.0 (1) | 59.1 (2) | Notes: (a) Asiri et al. (2010 ); (b) Sun et al. 2006 ); (c) Yan et al. 2006 ). | |
D—H⋯A | D—H | H⋯A | D⋯A | D—H⋯A | C11—H11B⋯O1i | 0.98 | 2.33 | 3.314 (2) | 177 | C12—H12⋯O1 | 0.95 | 2.32 | 3.028 (2) | 131 | C22—H22C⋯O1ii | 0.98 | 2.54 | 3.466 (2) | 157 | C7—H7⋯CgBiii | 0.95 | 2.79 | 3.674 (1) | 155 | Symmetry codes: (i) x, y+1, z; (ii) ; (iii) . | |
D—H⋯A | D—H | H⋯A | D⋯A | D—H⋯A | C11—H11A⋯O1i | 0.98 | 2.55 | 3.505 (2) | 165 | C12—H12A⋯O1 | 0.95 | 2.36 | 3.043 (2) | 128 | C14—H14⋯O1ii | 0.95 | 2.57 | 3.204 (2) | 124 | C17—H17⋯F1iii | 0.95 | 2.50 | 3.291 (2) | 141 | C7—H7⋯CgBiv | 0.95 | 2.90 | 3.608 (2) | 132 | Symmetry codes: (i) ; (ii) ; (iii) ; (iv) . | |
| Figure 1 A view of the molecular structure of I, with atom labelling. The displacement ellipsoids are drawn at the 50% probability level. |
| Figure 2 A view of the molecular structure of II, with atom labelling. The displacement ellipsoids are drawn at the 50% probability level. |
| Figure 3 A view of the structural overlap of compounds I (blue) and II (red); r.m.s. deviation 0.044 Å (Mercury; Macrae et al., 2020 ). The O, N and F atoms are shown as balls. |
The N1 and N2 nitrogen atoms of the pyrazole ring have pyramidal geometries (see Table 1
), with the sum of their bond angles being 350.5 (1) and 341.5 (1)°, respectively, in I, and 348.2 (1) and 351.0 (1)°, respectively, in II. The same pyramidal geometries of atoms N1 and N2 are also observed for the various analogues (Table 1
). The bond angles involving atoms N1 and N2 follow the same pattern.
4. Hirshfeld surface analysis and two-dimensional fingerprint plots
The Hirshfeld surface analyses and the associated two-dimensional fingerprint plots were performed with CrystalExplorer17 (Spackman et al., 2021
) following the protocol of Tan et al. (2019
). The Hirshfeld surfaces (HS) of I and TAYLUB01 are compared in Fig. 6
, and those for II and KELZIL and KEQXOU are compared in Fig. 7
. The large red spots indicate that short contacts are significant in the crystal packing of all five crystal structures. The full two-dimensional fingerprint plots for I and TAYLUB01, and for II and KELZIL and KEQXOU are given in Figs. 8
and 9
, respectively.
| Figure 6 The Hirshfeld surfaces of compounds, (a) I and (b) TAYLUB01 mapped over dnorm in the colour ranges −0.2834 to 1.4293 and −0.2505 to 1.2511 au., respectively. |
| Figure 7 The Hirshfeld surfaces of compounds, (a) II, (b) KELZIL and (c) KEQXOU, mapped over dnorm in the colour ranges −0.2048 to 1.21, −0.2236 to 1.3135 and −0.2367 to 1.3139 au., respectively. |
| Figure 8 The full two-dimensional fingerprint plots for compounds, (a) I and (b) TAYLUB01, and those delineated into H⋯H, C⋯H/H⋯C, N⋯H/H⋯N and O⋯H/H⋯O contacts. |
| Figure 9 The full two-dimensional fingerprint plots for compounds, (a) II, (b) KELZIL and (c) KEQXOU, and those delineated into H⋯H, C⋯H/H⋯C, N⋯H/H⋯N, O⋯H/H⋯O and halogen⋯H/H⋯halogen contacts. |
The contributions of the various inter-atomic contacts to the Hirshfeld surfaces for all five compounds are given in Table 5
. In I and TAYLUB01 the H⋯H contacts have a major contribution (60.6 and 57.7%, respectively) as do the C⋯H/H⋯C contributions (26.7 and 27.3%, respectively). These are followed by the O⋯H/H⋯O and N⋯H/H⋯N contributions (Table 5
). Other inter-atomic contacts, such as C⋯C and C⋯N/N⋯C contribute less than 2%. For II, KELZIL and KEQXOU the H⋯H contacts contribute ca 43% for all three compounds, notably less than in I and TAYLUB01. The contributions of the C⋯H/H⋯C, N⋯H/H⋯N and O⋯H/H⋯O contacts are similar to those for compound I (Table 5
). The halogen⋯H/H⋯halogen contributions vary from 10.5% in II to 13.5% in KEQXOU. The C⋯C contributions are 2.7, 2.0 and 2.2%, respectively, while the N⋯C/C⋯N contributions are 1.7, 2.0 and 2.2%, respectively. Both are more significant than for compound I and its analogue. The O⋯C/C⋯O contacts contribute less that 1%.
Contact | I | TAYLUB01a | II | KELZILb | KEQXOUc | | | | X = F | X = Cl | X = Br | H⋯H | 60.6 | 57.7 | 43.2 | 43.7 | 43.1 | C⋯H/H⋯C | 26.7 | 27.3 | 28.6 | 25.1 | 25.0 | N⋯H/H⋯N | 4.8 | 4.5 | 4.2 | 3.6 | 3.5 | O⋯H/H⋯O | 6.8 | 7.2 | 8.3 | 7.3 | 7.2 | X⋯H/H⋯X | – | – | 10.5 | 12.9 | 13.5 | C⋯C | 0.2 | 1.3 | 2.7 | 3.9 | 3.9 | N⋯C/C⋯N | 0.4 | 1.6 | 1.7 | 2.0 | 2.2 | O⋯C/C⋯O | 0 | 0 | 0.3 | 0.5 | 0.5 | X⋯X | – | – | 0.4 | 0.9 | 1.0 | Notes: (a) Asiri et al. (2010 ); (b) Sun et al. 2006 ); (c) Yan et al. 2006 ). | |
6. Database survey
A search of the CSD (CSD, Version 5.43, last update November 2022; Groom et al., 2016
) for benyzylidene-substituted 4-aminoantipyrine organic structures with R ≤ 0.05, no disorder, no ions, single-crystal analyses only gave more than 90 hits. In all compounds the configuration about the C=N bond is E. Various geometrical parameters of these compounds where analysed using Mercury (Macrae et al., 2020
). For example, the C=N bond lengths vary from 1.256 to 1.297 Å with a mean value of 1.281 Å (mean s.u. 0.008 Å). For compounds I and II and their analogues this bond length varies from 1.276 (2) Å for KELZIL (Sun et al., 2006
) to 1.291 (2) Å for I (see Table 1
), well within these limits. The C—N—N bond angles within the pyrazole ring vary from ca 107.7 to 110.7° with a mean value of 109.3° (mean s.u. 0.5°). The same angles in the title compounds (i.e. C1—N1—N2 and C3—N2—N1) and their analogues vary from 106.9 (3)° in KEQXOU (Yan et al., 2006
) to 109.58 (9)° in I for the former and 106.23 (9) in I to 107.7 (3)° in KEQXOU for the latter. The nitrogen atoms of the pyrazole ring have pyramidal geometries in all structures.
7. Synthesis, crystallization and spectroscopic analyses
Diethylaminobenzaldehyde (9.08 mmol, 1.744 g) and 1,5-dimethyl-2-phenyl-1H-pyrazol-3(2H)-one (9.08 mmol, 2.00 g) were added to 100 ml of methanol and the mixture was refluxed at 353 K for a period of 8 h. The solvent was then allowed to evaporate slowly at room temperature. Pale-yellow crystals of compound I were obtained after a period of three weeks. Melting point 492 K.
4-Fluorobenzaldehyde (9.80 mmol, 1.221 g) and 1,5-dimethyl-2-phenyl-1H-pyrazol-3(2H)-one (9.80 mol, 2.00 g) were added to 100 ml of methanol and the mixture was refluxed at 353 K for a period of 8 h. The solvent was then allowed to evaporate slowly at room temperature. Colourless crystals of compound II were obtained after a period of three weeks. Melting point 509 K.
The 1H NMR spectra of compounds I and II were recorded using a Bruker AC 400 MHz spectrometer (Fig. S3 in the supporting information). The compounds were dissolved in CDCl3 using tetramethylsilane as an internal standard and chemical shifts (δ) are stated in ppm. The imine proton resonated as a sharp singlet peak at 9.63 for I and at 9.73 for II, whereas the aromatic protons appeared as a multiplet at 6.69–7.74 for I and at 7.07–7.87 for II. The –NCH3 protons of the aminoantipyrine unit appeared as a singlet at 3.08 for I and 3.16 for II. The two ethyl [–N(CH2—CH3)2] group protons in the benzylidene moiety of compound I appeared as a multiplet at 1.09–1.32 and 3.42–3.45. The methyl protons (C—CH3) of the aminoantipyrine moiety appeared as a singlet at 2.49 for both I and II.
FT–IR spectra (KBr pellet) were recorded between 400 and 4000 cm−1 (Fig. S4 in the supporting information). The characteristic C=N stretching mode is observed at 1578 for I, and at 1577 cm−1 for II, confirming the formation of the Schiff base compounds. The weak band at 3037 (I) and 3035 cm−1 (II), is assigned to the aromatic C—H stretching vibration. The peaks observed at 1290–1010 (I) and 1294–1124 cm−1 (II) are due to the C—H in-plane bending vibration of the aromatic rings. The bands obtained at 753–976 (I) and 757–954 cm−1 (II) are assigned to C—H out-of-plane bending vibrations. The asymmetric and symmetric stretching vibrations of the methyl group in the 4-aminoantipyrine moiety are observed respectively in the ranges of 3010–2970 (I) and 2940–2900 cm−1 (II). The strong peaks at 1650 (I) and 1644 cm−1 (II) correspond to the carbonyl stretching vibrations.
8. Refinement
Crystal data, data collection and structure refinement details are summarized in Table 6
. The C-bound H atoms were included in calculated positions and treated as riding atoms: C—H = 0.95–1.0 Å with Uiso(H) = 1.5Ueq(C) for methyl H atoms and = 1.2Ueq(C) for other H atoms.
| I | II | Crystal data | Chemical formula | C22H26N4O | C18H16FN3O | Mr | 362.47 | 309.34 | Crystal system, space group | Monoclinic, C2/c | Orthorhombic, Pbca | Temperature (K) | 120 | 95 | a, b, c (Å) | 17.1588 (7), 7.0910 (3), 32.1594 (10) | 6.7886 (13), 16.6007 (3), 26.9563 (8) | α, β, γ (°) | 90, 102.338 (3), 90 | 90, 90, 90 | V (Å3) | 3822.6 (3) | 3037.9 (6) | Z | 8 | 8 | Radiation type | Cu Kα | Mo Kα | μ (mm−1) | 0.63 | 0.10 | Crystal size (mm) | 0.32 × 0.26 × 0.08 | 0.08 × 0.05 × 0.03 | | Data collection | Diffractometer | Xcalibur, Atlas, Gemini ultra | SuperNova, AtlasS2 | Absorption correction | Multi-scan (CrysAlis PRO; Agilent, 2010 ) | Multi-scan (CrysAlis PRO; Rigaku OD, 2022 ) | Tmin, Tmax | 0.273, 1.000 | 0.074, 1.000 | No. of measured, independent and observed [I > 2σ(I)] reflections | 15635, 3401, 2946 | 16735, 3041, 2346 | Rint | 0.034 | 0.064 | (sin θ/λ)max (Å−1) | 0.598 | 0.621 | | Refinement | R[F2 > 2σ(F2)], wR(F2), S | 0.036, 0.093, 1.06 | 0.042, 0.101, 1.05 | No. of reflections | 3401 | 3041 | No. of parameters | 248 | 211 | H-atom treatment | H-atom parameters constrained | H-atom parameters constrained | Δρmax, Δρmin (e Å−3) | 0.14, −0.21 | 0.21, −0.21 | Computer programs: CrysAlis PRO (Agilent, 2010 ), SUPERFLIP (Palatinus & Chapuis, 2007 ; JANA2006 (Petříček et al., 2014 ), PLATON (Spek, 2020 ); Mercury (Macrae et al., 2020 ), SHELXL2018/3 (Sheldrick, 2015 ) and publCIF (Westrip, 2010 ). | |
Supporting information
For both structures, data collection: CrysAlis PRO (Agilent, 2010); cell refinement: CrysAlis PRO (Agilent, 2010); data reduction: CrysAlis PRO (Agilent, 2010); program(s) used to solve structure: Superflip (Palatinus & Chapuis, 2007; JANA2006 (Petříček et al., 2014); program(s) used to refine structure: SHELXL2018/3 (Sheldrick, 2015); molecular graphics: PLATON (Spek, 2020) and Mercury (Macrae et al., 2020); software used to prepare material for publication: SHELXL2018/3 (Sheldrick, 2015), PLATON (Spek, 2020) and publCIF (Westrip, 2010).
(
E)-4-{[4-(Diethylamino)benzylidene]amino}-1,5-dimethyl-2-phenyl-1
H-pyrazol-3(2
H)-one (I)
top Crystal data top C22H26N4O | F(000) = 1552 |
Mr = 362.47 | Dx = 1.260 Mg m−3 |
Monoclinic, C2/c | Cu Kα radiation, λ = 1.5418 Å |
a = 17.1588 (7) Å | Cell parameters from 6730 reflections |
b = 7.0910 (3) Å | θ = 4.2–67.0° |
c = 32.1594 (10) Å | µ = 0.63 mm−1 |
β = 102.338 (3)° | T = 120 K |
V = 3822.6 (3) Å3 | Plate, yellow |
Z = 8 | 0.32 × 0.26 × 0.08 mm |
Data collection top Xcalibur, Atlas, Gemini ultra diffractometer | 3401 independent reflections |
Radiation source: Enhance Ultra (Cu) X-ray Source | 2946 reflections with I > 2σ(I) |
Mirror monochromator | Rint = 0.034 |
Detector resolution: 10.3784 pixels mm-1 | θmax = 67.1°, θmin = 5.3° |
ω scans | h = −20→20 |
Absorption correction: multi-scan (CrysAlisPro; Agilent, 2010) | k = −8→8 |
Tmin = 0.273, Tmax = 1.000 | l = −32→38 |
15635 measured reflections | |
Refinement top Refinement on F2 | Primary atom site location: structure-invariant direct methods |
Least-squares matrix: full | Secondary atom site location: difference Fourier map |
R[F2 > 2σ(F2)] = 0.036 | Hydrogen site location: inferred from neighbouring sites |
wR(F2) = 0.093 | H-atom parameters constrained |
S = 1.06 | w = 1/[σ2(Fo2) + (0.0498P)2 + 1.7059P] where P = (Fo2 + 2Fc2)/3 |
3401 reflections | (Δ/σ)max = 0.001 |
248 parameters | Δρmax = 0.14 e Å−3 |
0 restraints | Δρmin = −0.20 e Å−3 |
Special details top Geometry. All esds (except the esd in the dihedral angle between two l.s. planes) are estimated using the full covariance matrix. The cell esds are taken into account individually in the estimation of esds in distances, angles and torsion angles; correlations between esds in cell parameters are only used when they are defined by crystal symmetry. An approximate (isotropic) treatment of cell esds is used for estimating esds involving l.s. planes. |
Fractional atomic coordinates and isotropic or equivalent isotropic displacement parameters (Å2) top | x | y | z | Uiso*/Ueq | |
O1 | 0.95050 (6) | 0.16596 (12) | 0.13672 (3) | 0.0258 (2) | |
N1 | 0.91573 (6) | 0.42249 (14) | 0.17346 (3) | 0.0208 (2) | |
N2 | 0.95187 (6) | 0.59507 (14) | 0.18866 (3) | 0.0210 (2) | |
N3 | 1.09105 (6) | 0.43910 (15) | 0.12703 (3) | 0.0223 (2) | |
N4 | 1.37635 (7) | 0.11970 (15) | 0.04351 (4) | 0.0260 (3) | |
C1 | 0.96340 (7) | 0.32892 (17) | 0.14980 (4) | 0.0205 (3) | |
C2 | 1.02614 (7) | 0.46216 (17) | 0.14650 (4) | 0.0206 (3) | |
C3 | 1.01488 (7) | 0.61977 (17) | 0.16879 (4) | 0.0211 (3) | |
C4 | 0.87046 (7) | 0.32240 (16) | 0.19871 (4) | 0.0203 (3) | |
C5 | 0.89105 (7) | 0.32887 (17) | 0.24292 (4) | 0.0223 (3) | |
H5 | 0.933386 | 0.407554 | 0.256954 | 0.027* | |
C6 | 0.84902 (8) | 0.21906 (18) | 0.26626 (4) | 0.0242 (3) | |
H6 | 0.862682 | 0.222736 | 0.296476 | 0.029* | |
C7 | 0.78720 (8) | 0.10388 (17) | 0.24593 (4) | 0.0246 (3) | |
H7 | 0.759273 | 0.027492 | 0.262163 | 0.030* | |
C8 | 0.76628 (8) | 0.10055 (18) | 0.20181 (4) | 0.0256 (3) | |
H8 | 0.723541 | 0.022873 | 0.187820 | 0.031* | |
C9 | 0.80765 (8) | 0.21046 (18) | 0.17807 (4) | 0.0240 (3) | |
H9 | 0.793060 | 0.209089 | 0.147860 | 0.029* | |
C10 | 0.89517 (9) | 0.74834 (18) | 0.19053 (5) | 0.0283 (3) | |
H10A | 0.859334 | 0.711170 | 0.209086 | 0.042* | |
H10B | 0.863842 | 0.773905 | 0.161857 | 0.042* | |
H10C | 0.924493 | 0.862223 | 0.201903 | 0.042* | |
C11 | 1.06192 (8) | 0.79734 (18) | 0.17469 (4) | 0.0265 (3) | |
H11A | 1.083255 | 0.818394 | 0.205111 | 0.040* | |
H11B | 1.027446 | 0.903218 | 0.162966 | 0.040* | |
H11C | 1.106094 | 0.787545 | 0.159878 | 0.040* | |
C12 | 1.10770 (8) | 0.27332 (18) | 0.11476 (4) | 0.0230 (3) | |
H12 | 1.073861 | 0.170837 | 0.118039 | 0.028* | |
C13 | 1.17644 (8) | 0.23785 (18) | 0.09609 (4) | 0.0226 (3) | |
C14 | 1.19818 (8) | 0.05234 (19) | 0.08887 (4) | 0.0253 (3) | |
H14 | 1.166953 | −0.048700 | 0.095862 | 0.030* | |
C15 | 1.26374 (8) | 0.01199 (18) | 0.07186 (4) | 0.0249 (3) | |
H15 | 1.276951 | −0.115738 | 0.067713 | 0.030* | |
C16 | 1.31124 (8) | 0.15708 (18) | 0.06060 (4) | 0.0230 (3) | |
C17 | 1.28890 (8) | 0.34469 (18) | 0.06779 (4) | 0.0259 (3) | |
H17 | 1.319282 | 0.446570 | 0.060432 | 0.031* | |
C18 | 1.22408 (8) | 0.38183 (18) | 0.08523 (4) | 0.0242 (3) | |
H18 | 1.211242 | 0.509177 | 0.090042 | 0.029* | |
C19 | 1.40771 (8) | −0.07010 (18) | 0.04196 (4) | 0.0260 (3) | |
H19A | 1.395812 | −0.144034 | 0.065967 | 0.031* | |
H19B | 1.466432 | −0.063114 | 0.045749 | 0.031* | |
C20 | 1.37361 (9) | −0.1726 (2) | 0.00067 (4) | 0.0322 (3) | |
H20A | 1.396735 | −0.299257 | 0.001640 | 0.048* | |
H20B | 1.386511 | −0.102288 | −0.023225 | 0.048* | |
H20C | 1.315550 | −0.182293 | −0.003084 | 0.048* | |
C21 | 1.41662 (8) | 0.26801 (19) | 0.02481 (4) | 0.0267 (3) | |
H21A | 1.376832 | 0.363552 | 0.011689 | 0.032* | |
H21B | 1.440013 | 0.212963 | 0.001953 | 0.032* | |
C22 | 1.48234 (9) | 0.3647 (2) | 0.05712 (5) | 0.0325 (3) | |
H22A | 1.509447 | 0.457307 | 0.042542 | 0.049* | |
H22B | 1.520911 | 0.270251 | 0.071042 | 0.049* | |
H22C | 1.458950 | 0.428731 | 0.078544 | 0.049* | |
Atomic displacement parameters (Å2) top | U11 | U22 | U33 | U12 | U13 | U23 |
O1 | 0.0313 (5) | 0.0211 (4) | 0.0271 (5) | −0.0023 (4) | 0.0110 (4) | −0.0045 (3) |
N1 | 0.0240 (5) | 0.0178 (5) | 0.0219 (5) | −0.0019 (4) | 0.0078 (4) | −0.0020 (4) |
N2 | 0.0244 (5) | 0.0162 (5) | 0.0238 (5) | −0.0004 (4) | 0.0079 (4) | −0.0014 (4) |
N3 | 0.0229 (5) | 0.0243 (5) | 0.0202 (5) | 0.0009 (4) | 0.0058 (4) | 0.0007 (4) |
N4 | 0.0271 (6) | 0.0242 (6) | 0.0295 (6) | 0.0001 (4) | 0.0123 (5) | −0.0021 (4) |
C1 | 0.0241 (6) | 0.0201 (6) | 0.0175 (6) | 0.0028 (5) | 0.0050 (5) | 0.0013 (5) |
C2 | 0.0228 (6) | 0.0205 (6) | 0.0185 (6) | 0.0011 (5) | 0.0045 (5) | 0.0019 (5) |
C3 | 0.0229 (6) | 0.0203 (6) | 0.0201 (6) | 0.0018 (5) | 0.0045 (5) | 0.0036 (5) |
C4 | 0.0214 (6) | 0.0172 (6) | 0.0238 (6) | 0.0030 (5) | 0.0080 (5) | 0.0003 (5) |
C5 | 0.0208 (6) | 0.0218 (6) | 0.0244 (6) | −0.0002 (5) | 0.0051 (5) | −0.0028 (5) |
C6 | 0.0273 (7) | 0.0241 (6) | 0.0224 (6) | 0.0014 (5) | 0.0082 (5) | −0.0002 (5) |
C7 | 0.0247 (6) | 0.0209 (6) | 0.0305 (7) | 0.0004 (5) | 0.0109 (5) | 0.0013 (5) |
C8 | 0.0227 (6) | 0.0217 (6) | 0.0321 (7) | −0.0019 (5) | 0.0053 (5) | −0.0031 (5) |
C9 | 0.0250 (6) | 0.0239 (6) | 0.0225 (6) | 0.0002 (5) | 0.0035 (5) | −0.0019 (5) |
C10 | 0.0312 (7) | 0.0209 (6) | 0.0351 (7) | 0.0043 (5) | 0.0121 (6) | −0.0016 (5) |
C11 | 0.0304 (7) | 0.0205 (6) | 0.0295 (7) | −0.0026 (5) | 0.0084 (5) | 0.0001 (5) |
C12 | 0.0249 (6) | 0.0242 (6) | 0.0201 (6) | −0.0013 (5) | 0.0050 (5) | 0.0007 (5) |
C13 | 0.0239 (6) | 0.0260 (6) | 0.0179 (6) | 0.0006 (5) | 0.0044 (5) | −0.0012 (5) |
C14 | 0.0285 (7) | 0.0248 (6) | 0.0240 (6) | −0.0032 (5) | 0.0083 (5) | −0.0009 (5) |
C15 | 0.0296 (7) | 0.0211 (6) | 0.0249 (6) | 0.0004 (5) | 0.0078 (5) | −0.0027 (5) |
C16 | 0.0241 (6) | 0.0261 (6) | 0.0191 (6) | 0.0006 (5) | 0.0049 (5) | −0.0014 (5) |
C17 | 0.0277 (7) | 0.0234 (6) | 0.0284 (7) | −0.0024 (5) | 0.0099 (5) | −0.0010 (5) |
C18 | 0.0274 (7) | 0.0218 (6) | 0.0238 (6) | 0.0014 (5) | 0.0063 (5) | −0.0016 (5) |
C19 | 0.0258 (7) | 0.0272 (7) | 0.0261 (7) | 0.0032 (5) | 0.0078 (5) | −0.0001 (5) |
C20 | 0.0372 (8) | 0.0301 (7) | 0.0305 (7) | 0.0013 (6) | 0.0098 (6) | −0.0054 (6) |
C21 | 0.0296 (7) | 0.0267 (7) | 0.0268 (7) | −0.0008 (5) | 0.0126 (5) | −0.0017 (5) |
C22 | 0.0327 (7) | 0.0328 (7) | 0.0346 (7) | −0.0048 (6) | 0.0133 (6) | −0.0063 (6) |
Geometric parameters (Å, º) top O1—C1 | 1.2335 (15) | C11—H11A | 0.9800 |
N1—C1 | 1.3978 (16) | C11—H11B | 0.9800 |
N1—N2 | 1.4112 (14) | C11—H11C | 0.9800 |
N1—C4 | 1.4269 (16) | C12—C13 | 1.4551 (18) |
N2—C3 | 1.3796 (16) | C12—H12 | 0.9500 |
N2—C10 | 1.4685 (16) | C13—C18 | 1.3982 (18) |
N3—C12 | 1.2913 (17) | C13—C14 | 1.4002 (18) |
N3—C2 | 1.3991 (16) | C14—C15 | 1.3817 (19) |
N4—C16 | 1.3720 (17) | C14—H14 | 0.9500 |
N4—C19 | 1.4543 (17) | C15—C16 | 1.4073 (18) |
N4—C21 | 1.4569 (17) | C15—H15 | 0.9500 |
C1—C2 | 1.4533 (18) | C16—C17 | 1.4172 (18) |
C2—C3 | 1.3635 (17) | C17—C18 | 1.3737 (19) |
C3—C11 | 1.4858 (18) | C17—H17 | 0.9500 |
C4—C9 | 1.3881 (18) | C18—H18 | 0.9500 |
C4—C5 | 1.3907 (18) | C19—C20 | 1.5170 (19) |
C5—C6 | 1.3860 (18) | C19—H19A | 0.9900 |
C5—H5 | 0.9500 | C19—H19B | 0.9900 |
C6—C7 | 1.3868 (19) | C20—H20A | 0.9800 |
C6—H6 | 0.9500 | C20—H20B | 0.9800 |
C7—C8 | 1.3875 (19) | C20—H20C | 0.9800 |
C7—H7 | 0.9500 | C21—C22 | 1.523 (2) |
C8—C9 | 1.3877 (19) | C21—H21A | 0.9900 |
C8—H8 | 0.9500 | C21—H21B | 0.9900 |
C9—H9 | 0.9500 | C22—H22A | 0.9800 |
C10—H10A | 0.9800 | C22—H22B | 0.9800 |
C10—H10B | 0.9800 | C22—H22C | 0.9800 |
C10—H10C | 0.9800 | | |
| | | |
C1—N1—N2 | 109.58 (9) | H11A—C11—H11C | 109.5 |
C1—N1—C4 | 121.78 (10) | H11B—C11—H11C | 109.5 |
N2—N1—C4 | 119.11 (9) | N3—C12—C13 | 122.35 (12) |
C3—N2—N1 | 106.23 (9) | N3—C12—H12 | 118.8 |
C3—N2—C10 | 121.11 (10) | C13—C12—H12 | 118.8 |
N1—N2—C10 | 114.20 (10) | C18—C13—C14 | 116.99 (11) |
C12—N3—C2 | 119.50 (11) | C18—C13—C12 | 123.07 (11) |
C16—N4—C19 | 122.10 (11) | C14—C13—C12 | 119.93 (11) |
C16—N4—C21 | 121.63 (11) | C15—C14—C13 | 121.91 (12) |
C19—N4—C21 | 116.24 (10) | C15—C14—H14 | 119.0 |
O1—C1—N1 | 123.17 (11) | C13—C14—H14 | 119.0 |
O1—C1—C2 | 131.75 (11) | C14—C15—C16 | 121.06 (12) |
N1—C1—C2 | 105.07 (10) | C14—C15—H15 | 119.5 |
C3—C2—N3 | 123.15 (11) | C16—C15—H15 | 119.5 |
C3—C2—C1 | 107.70 (11) | N4—C16—C15 | 121.87 (11) |
N3—C2—C1 | 129.03 (11) | N4—C16—C17 | 121.24 (12) |
C2—C3—N2 | 110.64 (11) | C15—C16—C17 | 116.90 (12) |
C2—C3—C11 | 128.87 (12) | C18—C17—C16 | 121.17 (12) |
N2—C3—C11 | 120.44 (11) | C18—C17—H17 | 119.4 |
C9—C4—C5 | 120.63 (11) | C16—C17—H17 | 119.4 |
C9—C4—N1 | 118.27 (11) | C17—C18—C13 | 121.98 (12) |
C5—C4—N1 | 121.01 (11) | C17—C18—H18 | 119.0 |
C6—C5—C4 | 119.15 (12) | C13—C18—H18 | 119.0 |
C6—C5—H5 | 120.4 | N4—C19—C20 | 113.39 (11) |
C4—C5—H5 | 120.4 | N4—C19—H19A | 108.9 |
C5—C6—C7 | 120.64 (12) | C20—C19—H19A | 108.9 |
C5—C6—H6 | 119.7 | N4—C19—H19B | 108.9 |
C7—C6—H6 | 119.7 | C20—C19—H19B | 108.9 |
C6—C7—C8 | 119.79 (12) | H19A—C19—H19B | 107.7 |
C6—C7—H7 | 120.1 | C19—C20—H20A | 109.5 |
C8—C7—H7 | 120.1 | C19—C20—H20B | 109.5 |
C7—C8—C9 | 120.14 (12) | H20A—C20—H20B | 109.5 |
C7—C8—H8 | 119.9 | C19—C20—H20C | 109.5 |
C9—C8—H8 | 119.9 | H20A—C20—H20C | 109.5 |
C8—C9—C4 | 119.62 (12) | H20B—C20—H20C | 109.5 |
C8—C9—H9 | 120.2 | N4—C21—C22 | 113.00 (11) |
C4—C9—H9 | 120.2 | N4—C21—H21A | 109.0 |
N2—C10—H10A | 109.5 | C22—C21—H21A | 109.0 |
N2—C10—H10B | 109.5 | N4—C21—H21B | 109.0 |
H10A—C10—H10B | 109.5 | C22—C21—H21B | 109.0 |
N2—C10—H10C | 109.5 | H21A—C21—H21B | 107.8 |
H10A—C10—H10C | 109.5 | C21—C22—H22A | 109.5 |
H10B—C10—H10C | 109.5 | C21—C22—H22B | 109.5 |
C3—C11—H11A | 109.5 | H22A—C22—H22B | 109.5 |
C3—C11—H11B | 109.5 | C21—C22—H22C | 109.5 |
H11A—C11—H11B | 109.5 | H22A—C22—H22C | 109.5 |
C3—C11—H11C | 109.5 | H22B—C22—H22C | 109.5 |
| | | |
C1—N1—N2—C3 | 9.12 (13) | C4—C5—C6—C7 | 0.04 (19) |
C4—N1—N2—C3 | 156.03 (10) | C5—C6—C7—C8 | −1.04 (19) |
C1—N1—N2—C10 | 145.25 (10) | C6—C7—C8—C9 | 0.74 (19) |
C4—N1—N2—C10 | −67.83 (14) | C7—C8—C9—C4 | 0.54 (19) |
N2—N1—C1—O1 | 171.84 (11) | C5—C4—C9—C8 | −1.55 (18) |
C4—N1—C1—O1 | 25.96 (18) | N1—C4—C9—C8 | 175.24 (11) |
N2—N1—C1—C2 | −6.89 (13) | C2—N3—C12—C13 | −177.11 (11) |
C4—N1—C1—C2 | −152.77 (11) | N3—C12—C13—C18 | −7.96 (19) |
C12—N3—C2—C3 | 164.73 (12) | N3—C12—C13—C14 | 171.03 (12) |
C12—N3—C2—C1 | −10.89 (19) | C18—C13—C14—C15 | 0.13 (19) |
O1—C1—C2—C3 | −176.49 (13) | C12—C13—C14—C15 | −178.92 (12) |
N1—C1—C2—C3 | 2.09 (13) | C13—C14—C15—C16 | −0.7 (2) |
O1—C1—C2—N3 | −0.3 (2) | C19—N4—C16—C15 | −9.62 (19) |
N1—C1—C2—N3 | 178.24 (11) | C21—N4—C16—C15 | 168.66 (12) |
N3—C2—C3—N2 | −172.83 (11) | C19—N4—C16—C17 | 170.24 (12) |
C1—C2—C3—N2 | 3.60 (14) | C21—N4—C16—C17 | −11.49 (19) |
N3—C2—C3—C11 | 4.6 (2) | C14—C15—C16—N4 | −179.73 (12) |
C1—C2—C3—C11 | −178.95 (12) | C14—C15—C16—C17 | 0.41 (18) |
N1—N2—C3—C2 | −7.78 (13) | N4—C16—C17—C18 | −179.46 (12) |
C10—N2—C3—C2 | −140.20 (12) | C15—C16—C17—C18 | 0.40 (18) |
N1—N2—C3—C11 | 174.52 (11) | C16—C17—C18—C13 | −1.0 (2) |
C10—N2—C3—C11 | 42.11 (17) | C14—C13—C18—C17 | 0.71 (19) |
C1—N1—C4—C9 | −66.68 (16) | C12—C13—C18—C17 | 179.72 (12) |
N2—N1—C4—C9 | 150.54 (11) | C16—N4—C19—C20 | 92.75 (14) |
C1—N1—C4—C5 | 110.10 (13) | C21—N4—C19—C20 | −85.62 (14) |
N2—N1—C4—C5 | −32.67 (16) | C16—N4—C21—C22 | 88.73 (15) |
C9—C4—C5—C6 | 1.26 (18) | C19—N4—C21—C22 | −92.90 (14) |
N1—C4—C5—C6 | −175.45 (11) | | |
Hydrogen-bond geometry (Å, º) topCgB is the centroid of ring B (C4–C9). |
D—H···A | D—H | H···A | D···A | D—H···A |
C11—H11B···O1i | 0.98 | 2.33 | 3.314 (2) | 177 |
C12—H12···O1 | 0.95 | 2.32 | 3.028 (2) | 131 |
C22—H22C···O1ii | 0.98 | 2.54 | 3.466 (2) | 157 |
C7—H7···CgBiii | 0.95 | 2.79 | 3.674 (1) | 155 |
Symmetry codes: (i) x, y+1, z; (ii) x+1/2, y+1/2, z; (iii) −x+3/2, y−1/2, −z+1/2. |
(
E)-4-[(4-Fluorobenzylidene)amino]-1,5-dimethyl-2-phenyl-1
H-pyrazol-3(2
H)-one (II)
top Crystal data top C18H16FN3O | Dx = 1.353 Mg m−3 |
Mr = 309.34 | Mo Kα radiation, λ = 0.71073 Å |
Orthorhombic, Pbca | Cell parameters from 3075 reflections |
a = 6.7886 (13) Å | θ = 5.3–73.0° |
b = 16.6007 (3) Å | µ = 0.10 mm−1 |
c = 26.9563 (8) Å | T = 95 K |
V = 3037.9 (6) Å3 | Block, colourless |
Z = 8 | 0.08 × 0.05 × 0.03 mm |
F(000) = 1296 | |
Data collection top SuperNova, AtlasS2 diffractometer | 3041 independent reflections |
Radiation source: X-ray tube | 2346 reflections with I > 2σ(I) |
Mirror monochromator | Rint = 0.064 |
Detector resolution: 5.2027 pixels mm-1 | θmax = 26.2°, θmin = 1.5° |
ω scans | h = −7→8 |
Absorption correction: multi-scan (CrysAlisPro; Rigaku OD, 2022) | k = −20→20 |
Tmin = 0.074, Tmax = 1.000 | l = −21→33 |
16735 measured reflections | |
Refinement top Refinement on F2 | Secondary atom site location: difference Fourier map |
Least-squares matrix: full | Hydrogen site location: inferred from neighbouring sites |
R[F2 > 2σ(F2)] = 0.042 | H-atom parameters constrained |
wR(F2) = 0.101 | w = 1/[σ2(Fo2) + (0.0413P)2 + 0.3986P] where P = (Fo2 + 2Fc2)/3 |
S = 1.05 | (Δ/σ)max < 0.001 |
3041 reflections | Δρmax = 0.21 e Å−3 |
211 parameters | Δρmin = −0.21 e Å−3 |
0 restraints | Extinction correction: (SHELXL2018/3; Sheldrick, 2015), Fc*=kFc[1+0.001xFc2λ3/sin(2θ)]-1/4 |
Primary atom site location: structure-invariant direct methods | Extinction coefficient: 0.0025 (5) |
Special details top Geometry. All esds (except the esd in the dihedral angle between two l.s. planes) are estimated using the full covariance matrix. The cell esds are taken into account individually in the estimation of esds in distances, angles and torsion angles; correlations between esds in cell parameters are only used when they are defined by crystal symmetry. An approximate (isotropic) treatment of cell esds is used for estimating esds involving l.s. planes. |
Fractional atomic coordinates and isotropic or equivalent isotropic displacement parameters (Å2) top | x | y | z | Uiso*/Ueq | |
F1 | 0.87181 (18) | 0.01263 (7) | −0.22907 (4) | 0.0355 (3) | |
O1 | 0.72869 (16) | 0.08876 (7) | 0.07747 (4) | 0.0227 (3) | |
N1 | 0.45169 (19) | 0.15388 (8) | 0.10880 (5) | 0.0191 (3) | |
N2 | 0.28459 (19) | 0.19055 (8) | 0.08765 (5) | 0.0199 (3) | |
N3 | 0.48528 (19) | 0.10471 (8) | −0.02205 (5) | 0.0188 (3) | |
C1 | 0.5616 (2) | 0.11647 (10) | 0.07073 (6) | 0.0185 (3) | |
C2 | 0.4406 (2) | 0.12362 (9) | 0.02704 (6) | 0.0182 (3) | |
C3 | 0.2737 (2) | 0.16588 (9) | 0.03975 (6) | 0.0184 (3) | |
C4 | 0.5493 (2) | 0.19326 (10) | 0.14907 (6) | 0.0196 (3) | |
C5 | 0.5650 (2) | 0.27676 (11) | 0.15110 (6) | 0.0226 (4) | |
H5 | 0.504845 | 0.309414 | 0.126438 | 0.027* | |
C6 | 0.6700 (2) | 0.31165 (12) | 0.18982 (7) | 0.0274 (4) | |
H6 | 0.680539 | 0.368625 | 0.191832 | 0.033* | |
C7 | 0.7597 (2) | 0.26390 (13) | 0.22553 (7) | 0.0313 (4) | |
H7 | 0.832965 | 0.288135 | 0.251573 | 0.038* | |
C8 | 0.7421 (3) | 0.18061 (13) | 0.22317 (7) | 0.0300 (4) | |
H8 | 0.802971 | 0.147933 | 0.247699 | 0.036* | |
C9 | 0.6357 (2) | 0.14503 (11) | 0.18500 (6) | 0.0242 (4) | |
H9 | 0.622161 | 0.088118 | 0.183518 | 0.029* | |
C10 | 0.1160 (2) | 0.20736 (11) | 0.11996 (6) | 0.0240 (4) | |
H10A | 0.161622 | 0.234823 | 0.150023 | 0.036* | |
H10B | 0.051684 | 0.156623 | 0.129077 | 0.036* | |
H10C | 0.021908 | 0.241881 | 0.102403 | 0.036* | |
C11 | 0.0992 (2) | 0.18413 (11) | 0.00855 (7) | 0.0252 (4) | |
H11C | 0.124258 | 0.166697 | −0.025605 | 0.038* | |
H11B | 0.073898 | 0.242251 | 0.009018 | 0.038* | |
H11A | −0.015913 | 0.155503 | 0.021628 | 0.038* | |
C12 | 0.6468 (2) | 0.06772 (10) | −0.03275 (6) | 0.0196 (3) | |
H12A | 0.730837 | 0.049874 | −0.006810 | 0.024* | |
C13 | 0.7020 (2) | 0.05290 (9) | −0.08456 (6) | 0.0196 (3) | |
C14 | 0.8853 (3) | 0.01856 (10) | −0.09495 (6) | 0.0231 (4) | |
H14 | 0.970961 | 0.004617 | −0.068426 | 0.028* | |
C15 | 0.9441 (3) | 0.00454 (11) | −0.14364 (6) | 0.0257 (4) | |
H15 | 1.068284 | −0.019112 | −0.150837 | 0.031* | |
C16 | 0.8166 (3) | 0.02601 (11) | −0.18100 (6) | 0.0265 (4) | |
C17 | 0.6341 (3) | 0.06039 (11) | −0.17255 (6) | 0.0248 (4) | |
H17 | 0.550125 | 0.074561 | −0.199357 | 0.030* | |
C18 | 0.5772 (2) | 0.07358 (10) | −0.12379 (6) | 0.0222 (3) | |
H18 | 0.452267 | 0.096883 | −0.117004 | 0.027* | |
Atomic displacement parameters (Å2) top | U11 | U22 | U33 | U12 | U13 | U23 |
F1 | 0.0454 (6) | 0.0410 (7) | 0.0201 (5) | 0.0028 (5) | 0.0106 (5) | −0.0031 (4) |
O1 | 0.0174 (5) | 0.0287 (6) | 0.0220 (6) | 0.0060 (5) | −0.0013 (5) | −0.0022 (5) |
N1 | 0.0162 (6) | 0.0213 (7) | 0.0197 (6) | 0.0024 (5) | −0.0019 (5) | −0.0016 (5) |
N2 | 0.0145 (6) | 0.0222 (7) | 0.0231 (7) | 0.0029 (5) | −0.0011 (5) | −0.0002 (5) |
N3 | 0.0198 (6) | 0.0180 (7) | 0.0187 (6) | −0.0020 (5) | 0.0014 (5) | 0.0002 (5) |
C1 | 0.0189 (7) | 0.0171 (8) | 0.0196 (8) | −0.0015 (6) | 0.0023 (6) | 0.0002 (6) |
C2 | 0.0187 (7) | 0.0154 (7) | 0.0206 (8) | −0.0021 (6) | 0.0001 (6) | 0.0019 (6) |
C3 | 0.0181 (7) | 0.0161 (7) | 0.0211 (8) | −0.0032 (6) | −0.0003 (6) | 0.0011 (6) |
C4 | 0.0135 (7) | 0.0261 (9) | 0.0192 (8) | 0.0000 (6) | 0.0020 (6) | −0.0015 (6) |
C5 | 0.0187 (7) | 0.0266 (9) | 0.0223 (8) | −0.0009 (7) | 0.0020 (6) | −0.0011 (7) |
C6 | 0.0201 (8) | 0.0320 (10) | 0.0302 (9) | −0.0052 (7) | 0.0045 (7) | −0.0079 (8) |
C7 | 0.0190 (8) | 0.0497 (12) | 0.0251 (9) | −0.0032 (8) | −0.0009 (7) | −0.0106 (8) |
C8 | 0.0217 (8) | 0.0458 (12) | 0.0227 (8) | 0.0046 (8) | −0.0025 (7) | 0.0005 (8) |
C9 | 0.0187 (7) | 0.0310 (10) | 0.0229 (8) | 0.0023 (7) | 0.0027 (7) | 0.0008 (7) |
C10 | 0.0180 (7) | 0.0254 (9) | 0.0287 (9) | 0.0011 (6) | 0.0039 (7) | −0.0043 (7) |
C11 | 0.0220 (8) | 0.0251 (9) | 0.0284 (9) | 0.0035 (7) | −0.0046 (7) | −0.0002 (7) |
C12 | 0.0205 (7) | 0.0175 (8) | 0.0208 (8) | −0.0013 (6) | −0.0019 (6) | 0.0006 (6) |
C13 | 0.0227 (8) | 0.0151 (8) | 0.0210 (8) | −0.0023 (6) | 0.0011 (7) | 0.0005 (6) |
C14 | 0.0256 (8) | 0.0197 (8) | 0.0240 (8) | 0.0012 (7) | 0.0004 (7) | 0.0020 (6) |
C15 | 0.0269 (8) | 0.0218 (8) | 0.0284 (9) | 0.0030 (7) | 0.0062 (7) | −0.0005 (7) |
C16 | 0.0366 (9) | 0.0229 (9) | 0.0199 (8) | −0.0027 (7) | 0.0083 (7) | −0.0028 (6) |
C17 | 0.0285 (8) | 0.0257 (9) | 0.0202 (8) | −0.0013 (7) | −0.0014 (7) | 0.0002 (7) |
C18 | 0.0227 (8) | 0.0201 (8) | 0.0238 (8) | 0.0001 (6) | 0.0015 (7) | −0.0001 (6) |
Geometric parameters (Å, º) top F1—C16 | 1.367 (2) | C8—H8 | 0.9500 |
O1—C1 | 1.237 (2) | C9—H9 | 0.9500 |
N1—N2 | 1.4080 (18) | C10—H10A | 0.9800 |
N1—C1 | 1.413 (2) | C10—H10B | 0.9800 |
N1—C4 | 1.430 (2) | C10—H10C | 0.9800 |
N2—C3 | 1.356 (2) | C11—H11C | 0.9800 |
N2—C10 | 1.465 (2) | C11—H11B | 0.9800 |
N3—C12 | 1.289 (2) | C11—H11A | 0.9800 |
N3—C2 | 1.394 (2) | C12—C13 | 1.467 (2) |
C1—C2 | 1.441 (2) | C12—H12A | 0.9500 |
C2—C3 | 1.376 (2) | C13—C14 | 1.397 (2) |
C3—C11 | 1.484 (2) | C13—C18 | 1.398 (2) |
C4—C9 | 1.387 (2) | C14—C15 | 1.392 (2) |
C4—C5 | 1.391 (2) | C14—H14 | 0.9500 |
C5—C6 | 1.390 (2) | C15—C16 | 1.375 (3) |
C5—H5 | 0.9500 | C15—H15 | 0.9500 |
C6—C7 | 1.388 (3) | C16—C17 | 1.383 (3) |
C6—H6 | 0.9500 | C17—C18 | 1.387 (2) |
C7—C8 | 1.389 (3) | C17—H17 | 0.9500 |
C7—H7 | 0.9500 | C18—H18 | 0.9500 |
C8—C9 | 1.389 (3) | | |
| | | |
N2—N1—C1 | 108.75 (12) | N2—C10—H10A | 109.5 |
C1—N1—C4 | 120.50 (13) | N2—C10—H10B | 109.5 |
N2—N1—C4 | 118.90 (13) | H10A—C10—H10B | 109.5 |
C3—N2—N1 | 107.40 (13) | N2—C10—H10C | 109.5 |
C3—N2—C10 | 125.50 (14) | H10A—C10—H10C | 109.5 |
N1—N2—C10 | 118.09 (13) | H10B—C10—H10C | 109.5 |
C12—N3—C2 | 120.32 (14) | C3—C11—H11C | 109.5 |
O1—C1—N1 | 122.75 (15) | C3—C11—H11B | 109.5 |
O1—C1—C2 | 132.33 (15) | H11C—C11—H11B | 109.5 |
N1—C1—C2 | 104.85 (13) | C3—C11—H11A | 109.5 |
C3—C2—N3 | 122.05 (14) | H11C—C11—H11A | 109.5 |
C3—C2—C1 | 107.94 (14) | H11B—C11—H11A | 109.5 |
N3—C2—C1 | 129.30 (14) | N3—C12—C13 | 120.68 (15) |
N2—C3—C2 | 110.24 (14) | N3—C12—H12A | 119.7 |
N2—C3—C11 | 121.43 (14) | C13—C12—H12A | 119.7 |
C2—C3—C11 | 128.33 (15) | C14—C13—C18 | 119.24 (15) |
C9—C4—C5 | 121.03 (16) | C14—C13—C12 | 119.14 (15) |
C9—C4—N1 | 117.53 (15) | C18—C13—C12 | 121.62 (15) |
C5—C4—N1 | 121.38 (15) | C15—C14—C13 | 120.84 (16) |
C6—C5—C4 | 118.93 (16) | C15—C14—H14 | 119.6 |
C6—C5—H5 | 120.5 | C13—C14—H14 | 119.6 |
C4—C5—H5 | 120.5 | C16—C15—C14 | 117.85 (16) |
C7—C6—C5 | 120.50 (18) | C16—C15—H15 | 121.1 |
C7—C6—H6 | 119.7 | C14—C15—H15 | 121.1 |
C5—C6—H6 | 119.7 | F1—C16—C15 | 118.67 (16) |
C6—C7—C8 | 119.95 (17) | F1—C16—C17 | 117.94 (16) |
C6—C7—H7 | 120.0 | C15—C16—C17 | 123.39 (16) |
C8—C7—H7 | 120.0 | C16—C17—C18 | 118.07 (16) |
C9—C8—C7 | 120.12 (18) | C16—C17—H17 | 121.0 |
C9—C8—H8 | 119.9 | C18—C17—H17 | 121.0 |
C7—C8—H8 | 119.9 | C17—C18—C13 | 120.62 (16) |
C4—C9—C8 | 119.45 (18) | C17—C18—H18 | 119.7 |
C4—C9—H9 | 120.3 | C13—C18—H18 | 119.7 |
C8—C9—H9 | 120.3 | | |
| | | |
C1—N1—N2—C3 | 9.45 (17) | N2—N1—C4—C5 | −36.0 (2) |
C4—N1—N2—C3 | 152.54 (14) | C1—N1—C4—C5 | 102.73 (18) |
C1—N1—N2—C10 | 158.44 (14) | C9—C4—C5—C6 | 0.3 (2) |
C4—N1—N2—C10 | −58.47 (19) | N1—C4—C5—C6 | −176.80 (14) |
N2—N1—C1—O1 | 170.21 (15) | C4—C5—C6—C7 | 0.7 (2) |
C4—N1—C1—O1 | 27.8 (2) | C5—C6—C7—C8 | −1.0 (3) |
N2—N1—C1—C2 | −7.02 (17) | C6—C7—C8—C9 | 0.3 (3) |
C4—N1—C1—C2 | −149.42 (14) | C5—C4—C9—C8 | −1.0 (2) |
C12—N3—C2—C3 | 177.33 (15) | N1—C4—C9—C8 | 176.19 (14) |
C12—N3—C2—C1 | 8.2 (3) | C7—C8—C9—C4 | 0.7 (2) |
O1—C1—C2—C3 | −174.69 (18) | C2—N3—C12—C13 | −175.43 (14) |
N1—C1—C2—C3 | 2.15 (17) | N3—C12—C13—C14 | 174.82 (15) |
O1—C1—C2—N3 | −4.3 (3) | N3—C12—C13—C18 | −4.0 (2) |
N1—C1—C2—N3 | 172.51 (15) | C18—C13—C14—C15 | −0.3 (2) |
N1—N2—C3—C2 | −8.11 (18) | C12—C13—C14—C15 | −179.17 (16) |
C10—N2—C3—C2 | −154.17 (15) | C13—C14—C15—C16 | 0.4 (3) |
N1—N2—C3—C11 | 171.33 (14) | C14—C15—C16—F1 | −179.96 (16) |
C10—N2—C3—C11 | 25.3 (2) | C14—C15—C16—C17 | −0.2 (3) |
N3—C2—C3—N2 | −167.49 (14) | F1—C16—C17—C18 | 179.62 (15) |
C1—C2—C3—N2 | 3.71 (18) | C15—C16—C17—C18 | −0.2 (3) |
N3—C2—C3—C11 | 13.1 (3) | C16—C17—C18—C13 | 0.3 (3) |
C1—C2—C3—C11 | −175.67 (16) | C14—C13—C18—C17 | −0.1 (2) |
N2—N1—C4—C9 | 146.82 (14) | C12—C13—C18—C17 | 178.78 (15) |
C1—N1—C4—C9 | −74.49 (19) | | |
Hydrogen-bond geometry (Å, º) topCgB is the centroid of ring B (C4–C9). |
D—H···A | D—H | H···A | D···A | D—H···A |
C11—H11A···O1i | 0.98 | 2.55 | 3.505 (2) | 165 |
C12—H12A···O1 | 0.95 | 2.36 | 3.043 (2) | 128 |
C14—H14···O1ii | 0.95 | 2.57 | 3.204 (2) | 124 |
C17—H17···F1iii | 0.95 | 2.50 | 3.291 (2) | 141 |
C7—H7···CgBiv | 0.95 | 2.90 | 3.608 (2) | 132 |
Symmetry codes: (i) x−1, y, z; (ii) −x+2, −y, −z; (iii) x−1/2, y, −z−1/2; (iv) x+1/2, y, −z+1/2. |
Selected geometric parameters (Å, °) for I and TAYLUB01a, and for II and KELZILb and KEQXOUc top | I | TAYLUB01a | II | KELZILb | KEQXOUc |
N3—C12 | 1.291 (2) | 1.288 (2) | 1.289 (2) | 1.276 (2) | 1.279 (5) |
C2—N3—C12—C13 | –177.11 (11) | 173.20 (11) | –175.43 (14) | –176.68 (15) | 177.5 (4) |
| | | | | |
C1—N1—N2 | 109.58 (9) | 109.58 (10) | 108.75 (12) | 108.58 (13) | 106.9 (3) |
C1—N1—C4 | 121.78 (10) | 122.30 (10) | 120.50 (13) | 122.40 (13) | 122.4 (3) |
N2—N1—C4 | 119.11 (9) | 118.13 (10) | 118.90 (13) | 119.12 (14) | 119.8 (3) |
Sum | 350.47 (9) | 350.0 (1) | 348.15 (13) | 350.10 (13) | 349.1 (3) |
| | | | | |
C3—N2—N1 | 106.23 (9) | 106.50 (10) | 107.40 (13) | 107.34 (13) | 107.7 (3) |
C3—N2—C10 | 121.11 (10) | 122.30 (11) | 125.50 (14) | 124.77 (14) | 125.1 (3) |
N1—N2—C10 | 114.20 (10) | 114.68 (10) | 118.09 (13) | 117.05 (15) | 115.9 (3) |
Sum | 341.54 (10) | 343.48 (10) | 350.99 (13) | 349.16 (14) | 348.7 (3) |
Notes: (a) Asiri et al. (2010); (b) Sun et al. 2006); (c) Yan et al. 2006). |
A comparison of various dihedral angles (°) for I and TAYLUB01a, and for II and KELZILb and KEQXOUc topA = ring N1/N2/C1–C3, B = ring C4–C9, C = ring C13–C18 (atom numbering following this paper). |
Dihedral angle | I | TAYLUB01a | II | KELZILb | KEQXOUc |
Planes A to B | 54.87 (7) | 55.01 (7) | 60.44 (8) | 51.6 (1) | 50.8 (2) |
Planes A to C | 22.92 (7) | 19.03 (7) | 12.70 (9) | 8.7 (1) | 9.1 (2) |
Planes B to C | 73.98 (6) | 73.98 (6) | 71.28 (8) | 59.0 (1) | 59.1 (2) |
Notes: (a) Asiri et al. (2010); (b) Sun et al. 2006); (c) Yan et al. 2006). |
Principal percentage contributions of inter-atomic contacts to the Hirshfeld surfaces of I, TAYLUB01a, II, KELZILb and KEQXOUc topContact | I | TAYLUB01a | II | KELZILb | KEQXOUc |
| | | X = F | X = Cl | X = Br |
H···H | 60.6 | 57.7 | 43.2 | 43.7 | 43.1 |
C···H/H···C | 26.7 | 27.3 | 28.6 | 25.1 | 25.0 |
N···H/H···N | 4.8 | 4.5 | 4.2 | 3.6 | 3.5 |
O···H/H···O | 6.8 | 7.2 | 8.3 | 7.3 | 7.2 |
X···H/H···X | – | – | 10.5 | 12.9 | 13.5 |
C···C | 0.2 | 1.3 | 2.7 | 3.9 | 3.9 |
N···C/C···N | 0.4 | 1.6 | 1.7 | 2.0 | 2.2 |
O···C/C···O | 0 | 0 | 0.3 | 0.5 | 0.5 |
X···X | – | – | 0.4 | 0.9 | 1.0 |
Notes: (a) Asiri et al. (2010); (b) Sun et al. 2006); (c) Yan et al. 2006). |
Acknowledgements
MGS and AS thank the Central Instrumentation Facility, Pondicherry University and STIC, Cochin, for the use of their facilities. MK and MD acknowledge using the CzechNanoLab Research Infrastructure supported by MEYS CR (No. LM2018110) for crystallographic analysis. HSE is grateful to the University of Neuchâtel for their support over the years.
References
Abd El Rehim, S. S., Ibrahim, M. A. M. & Khalid, K. F. (2001). Mater. Chem. Phys. 70, 268–273. Web of Science CrossRef CAS Google Scholar
Agilent (2010). CrysAlis PRO. Agilent Technologies, Yarnton, England. Google Scholar
Alam, M. S. & Lee, D.-U. (2016). EXCLI J. 15, 614–629. Web of Science PubMed Google Scholar
Asiri, A. M., Khan, S. A., Tan, K. W. & Ng, S. W. (2010). Acta Cryst. E66, o1751. Web of Science CSD CrossRef IUCr Journals Google Scholar
Bashkatova, N. V., Korotkova, E. I., Karbainov, Y. A., Yagovkin, A. Y. & Bakibaev, A. A. (2005). J. Pharm. Biomed. Anal. 37, 1143–1147. Web of Science CrossRef PubMed CAS Google Scholar
Collado, M. S., Mantovani, V. E., Goicoechea, H. C. & Olivieri, A. C. (2000). Talanta, 52, 909–920. Web of Science CrossRef PubMed CAS Google Scholar
Groom, C. R., Bruno, I. J., Lightfoot, M. P. & Ward, S. C. (2016). Acta Cryst. B72, 171–179. Web of Science CrossRef IUCr Journals Google Scholar
Macrae, C. F., Sovago, I., Cottrell, S. J., Galek, P. T. A., McCabe, P., Pidcock, E., Platings, M., Shields, G. P., Stevens, J. S., Towler, M. & Wood, P. A. (2020). J. Appl. Cryst. 53, 226–235. Web of Science CrossRef CAS IUCr Journals Google Scholar
Nibila, T. A., Shameera Ahamed, T. K., Soufeena, P. P., Muraleedharan, K., Peiyat, P. & Aravindakshan, K. K. (2020). Results Chem. 2, 100062. https://doi.org/10.1016/j.rechem.2020.100062 Google Scholar
Oudar, J. L. (1977). J. Chem. Phys. 67, 446–457. CrossRef CAS Web of Science Google Scholar
Palatinus, L. & Chapuis, G. (2007). J. Appl. Cryst. 40, 786–790. Web of Science CrossRef CAS IUCr Journals Google Scholar
Petříček, V., Dušek, M. & Palatinus, L. (2014). Z. Kristallogr. 229, 345–352. Google Scholar
Raman, N., Dhaveethu Raja, J. & Sakthivel, A. (2007). J. Chem. Sci. 119, 303–310. Web of Science CrossRef CAS Google Scholar
Rigaku OD (2022). CrysAlis PRO. Rigaku Oxford Diffraction Ltd, Yarnton, England. Google Scholar
Senthilkumar, K., Thirumoorthy, K., Dragonetti, C., Marinotto, D., Righetto, S., Colombo, A., Haukka, M. & Palanisami, N. (2016). Dalton Trans. 45, 11939–11943. Web of Science CSD CrossRef CAS PubMed Google Scholar
Sheldrick, G. M. (2015). Acta Cryst. C71, 3–8. Web of Science CrossRef IUCr Journals Google Scholar
Spackman, P. R., Turner, M. J., McKinnon, J. J., Wolff, S. K., Grimwood, D. J., Jayatilaka, D. & Spackman, M. A. (2021). J. Appl. Cryst. 54, 1006–1011. Web of Science CrossRef CAS IUCr Journals Google Scholar
Spek, A. L. (2020). Acta Cryst. E76, 1–11. Web of Science CrossRef IUCr Journals Google Scholar
Sun, Y.-X., Zhang, R., Jin, Q.-M., Zhi, X.-J. & Lü, X.-M. (2006). Acta Cryst. C62, o467–o469. Web of Science CSD CrossRef CAS IUCr Journals Google Scholar
Tan, S. L., Jotani, M. M. & Tiekink, E. R. T. (2019). Acta Cryst. E75, 308–318. Web of Science CrossRef IUCr Journals Google Scholar
Westrip, S. P. (2010). J. Appl. Cryst. 43, 920–925. Web of Science CrossRef CAS IUCr Journals Google Scholar
Yan, G.-B., Zheng, Y.-F., Zhang, C.-N. & Yang, M.-H. (2006). Acta Cryst. E62, o5328–o5329. Web of Science CSD CrossRef IUCr Journals Google Scholar
Zyss, J. (1979). J. Chem. Phys. 70, 3341–3349. CrossRef CAS Web of Science Google Scholar
This is an open-access article distributed under the terms of the Creative Commons Attribution (CC-BY) Licence, which permits unrestricted use, distribution, and reproduction in any medium, provided the original authors and source are cited.
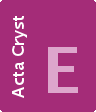 | CRYSTALLOGRAPHIC COMMUNICATIONS |
ISSN: 2056-9890
Open

access