1. Chemical context
The synthesis and physical characterization of the title compound, 1-chloro-4-[2-(4-chlorophenyl)ethyl]benzene, C14H12Cl2, (I)
, has been reported by several research groups over the years (Otsubo et al., 1980
; Bestiuc et al., 1985
; Parnes et al., 1989
; Hu et al., 2011
; Liu & Li, 2007
). In the same way, the bromo analogue of (I)
, 1-bromo-4-[2-(4-bromophenyl)ethyl]benzene, C14H12Br2, (II)
, has been described previously (Golden, 1961
; Otsubo et al., 1980
; Remizov et al., 2005
; Liu & Li, 2007
). Despite this interest, crystallographic characterization is lacking. Recently, compounds (I)
and (II)
became available as minor side-products during the synthesis of the respective tri(4-halobenzyl)tin hydroxide from the reaction of tri(4-halobenzyl)tin halide and sodium hydroxide. Herein, the crystal and molecular structures of (I)
and (II)
are described. The structures are not isostructural and in order to gain further insight into the molecular packing, the structures were subjected to an analysis of their Hirshfeld surfaces along with some computational chemistry.
2. Structural commentary
The two independent molecules comprising the asymmetric unit of (I)
are shown in Fig. 1
(a) and (b); each is disposed about a centre of inversion. The molecules present very similar features and, from inversion symmetry, comprise parallel benzene rings. The C3A—C4A—C7A—C7Ai and C5A—C4A—C7A—C7Ai torsion angles of −83.46 (19) and 95.17 (17)° highlight deviations from mirror symmetry in the molecule [symmetry operation: (i) 1 − x, 1 − y, 1 − z]. These values are equal within experimental error and are very close to the equivalent angles for the second independent molecule of −83.7 (2) and 94.75 (19)°, respectively [symmetry operation: (ii)
− x,
− y, 1 − z].
| Figure 1 The molecular structures of (a) the Cl1A-containing molecule of (I) , (b) the Cl1B-containing molecule of (I) and (c) the molecule of (II) showing the atom-labelling scheme and displacement ellipsoids at the 70% probability level. Unlabelled atoms in (a) and (b) are related by the symmetry operations 1 − x, 1 − y, 1 − z and − x, − y, 1 − z, respectively. |
The molecule of (II)
is shown in Fig. 1
(c) and does not feature the molecular symmetry of (I)
. The difference in the conformation in (II)
, cf. (I)
, is seen immediately in the magnitude of the dihedral angle formed between the benzene rings of 59.29 (11)°, indicating an inclined disposition. The central torsion angle, i.e. C4—C7—C8—C9 of 172.1 (2)°, deviates from the 180° angles observed for the two independent molecules in (I)
. The twist in the molecule of (II)
is reflected in the four torsion angles C3—C4—C7—C8 [46.6 (3)°], C5—C4—C7—C8 [−134.8 (2)°], C7—C8—C9—C14 [16.4 (3)°] and C7—C8—C9—C10 [−163.7 (2)°].
The conformational differences between the molecules in (I)
and (II)
are highlighted in the overlay diagram shown in Fig. 2
.
| Figure 2 Overlap diagram of the (a) Cl1A-molecule in (I) (red image), (b) Cl1B-molecule in (I) (green) and (c) the molecule in (II) (blue). Molecules have been overlapped so that the C1-benzene rings are coincident. |
3. Supramolecular features
In the crystal of (I)
, the main point of contact between the independent molecules comprising the asymmetric unit are of the type benzene-C—H⋯π(benzene), Table 1
. The result is the formation of a supramolecular chain along the a-axis direction. Chains are connected into a supramolecular layer via end-on Cl1A⋯Cl1Aiii contacts [3.3184 (7) Å and C1—C11A⋯Cl1Aiii = 164.61 (5)° for symmetry operation (iii)
− x,
− y, −z], Fig. 3
(a). The topology of the layer is flat and connections between the layers that stack along [1
0] are weaker end-on Cl1B⋯Cl1Biv contacts [3.4322 (7) Å and C1B—Cl1B⋯Cl1Biv = 155.19 (5)° for symmetry operation (iv) 1 − x, 2 − y, 2 − z], which lead to a three-dimensional (3-D) architecture. As seen from Fig, 3(b), there are large voids defined by the aforementioned contacts which enables twofold, 3D–3D interpenetration, Fig. 3
(c).
D—H⋯A | D—H | H⋯A | D⋯A | D—H⋯A | C5B—H5B⋯Cg1 | 0.93 | 2.62 | 3.4866 (15) | 155 | | |
| Figure 3 Molecular packing in (I) : (a) a view of the supramolecular layer parallel to [1 0] sustained by C—H⋯π and Cl⋯Cl contacts shown as purple and orange dashed lines, respectively, (b) a view of half of the unit-cell contents shown in projection down the c axis and (c) an image highlighting the twofold interpenetration in space-filling mode. |
The 3-D architecture of (II)
is supported by benzene-C—H⋯π(benzene) and Br⋯Br contacts. Globally, molecules assemble in the ac plane and are connected to layers along [010] by benzene-C—H⋯π(benzene) contacts, Table 2
. Further, lateral interactions are Br1⋯Br2i [3.5242 (4) Å, C1—Br⋯Br2i = 144.67 (7)° and C12i—Br2i⋯Br1 = 154.39 (7)° for symmetry operation (i) 1 + x, y, 1 + z; Fig. 4
].
D—H⋯A | D—H | H⋯A | D⋯A | D—H⋯A | C3—H3⋯Cg2i | 0.95 | 2.69 | 3.442 (2) | 136 | C6—H6⋯Cg1ii | 0.95 | 2.91 | 3.704 (2) | 141 | C13—H13⋯Cg2iii | 0.95 | 2.87 | 3.569 (2) | 131 | Symmetry codes: (i) -x, -y+1, -z+1; (ii) ; (iii) . | |
| Figure 4 Molecular packing in (II) : a view of the unit-cell contents shown in projection down the a axis, highlighting C—H⋯π and Br⋯Br contacts as purple and orange dashed lines, respectively. |
4. Hirshfeld surface analysis
The Hirshfeld surface calculations for (I)
and (II)
were performed in accord with established procedures (Tan et al., 2019
) with the aid of Crystal Explorer (Turner et al., 2017
) to determine the influence of weak intermolecular interactions upon the molecular packing in the absence of conventional hydrogen bonds.
In the crystal of (I)
, with two independent molecules, labelled A and B, disposed about a centre of inversion the presence of faint-red spots near the benzene-C2A, C3A and H5B atoms in the images of Hirshfeld surfaces mapped over dnorm in Fig. 5
represent C—H⋯π contacts, Tables 1
and 3
. The diminutive red spot viewed near the benzene-C5B atom in Fig. 5
(b) indicates the effect of a short interatomic C5B⋯H2B contact, Table 3
. Also, the presence of diminutive red spots near the terminal chlorine atoms of both independent molecules in Fig. 5
are due to the formation of short interatomic Cl⋯Cl contacts, Table 3
.
Contact | Distance | Symmetry operation | (I) | | | H6B⋯H72A | 2.35 | x, y, z | H5B⋯C2A | 2.75 | x, y, z | H5B⋯C3A | 2.72 | x, y, z | H2B⋯C5B | 2.67 | x, 2 − y, + z | C11A⋯Cl1A | 3.3184 (7) | − x, − y, −z | Cl1B⋯Cl1B | 3.4322 (7) | 1 − x, 2 − y, 2 − z | (II) | | | H8B⋯H8B | 2.21 | −x, 2 − y, 1 − z | H3⋯C13 | 2.74 | −x, 1 − y, 1 − z | H3⋯C14 | 2.72 | −x, 1 − y, 1 − z | H6⋯C1 | 2.82 | 1 − x, + y, − z | H6⋯C2 | 2.62 | 1 − x, + y, − z | H11⋯C6 | 2.80 | −x, 2 − y, 1 − z | Br1⋯Br2 | 3.5242 (4) | 1 + x, y, 1 + z | Notes: (a) The interatomic distances are calculated in Crystal Explorer (Turner et al., 2017 ) whereby the X—H bond lengths are adjusted to their neutron values. | |
| Figure 5 Views of the Hirshfeld surfaces for (I) mapped over dnorm for (a) molecule A [in the range −0.103 to +1.259 arbitrary units] and (b) molecule B [−0.072 to +1.234 arbitrary units] highlighting the short interatomic C⋯H/H⋯C and H⋯H contacts through black and red dashed lines, respectively. |
In the crystal of (II)
, the bright-red spots near the bromine atoms on the Hirshfeld surfaces mapped over dnorm in Fig. 6
indicate interatomic Br⋯Br contacts, Table 3
, whereas those near the benzene-C2 and H6 atoms in Fig. 6
(b) indicate short interatomic C—H⋯π interactions, Table 3
. The presence of faint-red spots near the benzene-C13, C14 and H3 atoms in Fig. 6
(a) also reflect the presence of C—H⋯π contacts, Table 3
.
| Figure 6 Views of the Hirshfeld surfaces for (II) mapped over dnorm [in the range −0.104 to +1.172 arbitrary units] highlighting the short interatomic C⋯H/H⋯C contacts through black dashed lines. |
From the views of Hirshfeld surfaces mapped over the calculated electrostatic potentials in Figs. 7
(a) and (b) for the independent molecules of (I)
highlight the small deviations from putative mirror symmetry through the slight differences in the blue and red regions around the atoms of their surfaces corresponding, respectively, to positive and negative potentials. For (II)
, Fig.7(c), the donors and acceptors of the C—H⋯π interactions are viewed as blue bumps and light-red concave regions. Further, the donors and acceptors of the C—H⋯π contacts for each of (I)
and (II)
are also illustrated through black dotted lines on the Hirshfeld surfaces mapped with shape-index properties in Fig. 8
.
| Figure 7 Views of the Hirshfeld surfaces mapped over the calculated electrostatic potential for (a) (I) , molecule A [−0.032 to +0.035 a.u.], (b) (I) , molecule B in [−0.033 to +0.044 a.u.] range and (c) (II) [−0.022 to +0.039 a.u.]. The red and blue regions represent negative and positive electrostatic potentials, respectively. |
| Figure 8 Views of the Hirshfeld surfaces mapped with the shape index property for (a) (I) , molecule B, (b)–(d) (II) , highlighting intermolecular C—H⋯π interactions through black dotted lines. |
The overall two-dimensional fingerprint plot for the independent molecules A and B as well as entire (I)
are shown in Fig. 9
(a), and those delineated into H⋯H, C⋯H/H⋯C, Cl⋯H/H⋯Cl and Cl⋯Cl contacts are illustrated in Fig. 9
(b)–(e), respectively. The quantitative summary of percentage contributions from the different interatomic contacts to the respective Hirshfeld surfaces of A, B and (I)
are presented in Table 4
.
Contact | Percentage contribution | | | | | (I) - molecule A | (I) - molecule B | (I) | (II) | H⋯H | 30.8 | 35.1 | 31.4 | 30.6 | C⋯H/H⋯C | 32.5 | 27.0 | 28.4 | 32.7 | X⋯H/H⋯X | 30.5 | 33.3 | 34.2 | 30.4 | X⋯X | 3.9 | 2.2 | 3.4 | 4.9 | C⋯C | 1.3 | 1.3 | 1.4 | 0.0 | C⋯X/X⋯C | 1.1 | 1.1 | 1.2 | 1.4 | | |
| Figure 9 (a) The full two-dimensional fingerprint plot for molecule A of (I) , molecule B of (I) , and overall (I) , and (b)–(e) those delineated into H⋯H, C⋯H/H⋯C, Cl⋯H/H⋯Cl and Cl⋯Cl contacts. |
Some qualitative differences in the fingerprint plots are evident for molecules A and B, confirming their distinct packing interactions. The complementary pair of forceps-like tips at de + di ∼2.3 Å in the fingerprint plots delineated into H⋯H contacts for A and B in Fig. 9
(b) represent the short interatomic H⋯H contact, Table 3
, which merge to form a pair of tips in the overall plot for (I)
. The fingerprint plots delineated into C⋯H/H⋯C contacts for molecules A and B in Fig. 9
(c) exhibit the clearest distinction between the interatomic contacts formed by the molecules through the asymmetric distribution of points. The complementary distribution of points in the acceptor and donor regions of the plots for A and B, respectively, with the peaks at de + di ∼2.7 Å, are due to the formation of short interatomic C⋯H/H⋯C contacts between the benzene-C2A, C3A and H5B atoms, Table 3
. Similar short interatomic contacts between benzene-C5B and H2B atoms of B results in forceps-like tips at de + di ∼2.7 Å in the acceptor region of the plot whereas it is merged within the tip of previously mentioned contact in the donor region. However, the respective plot for an overall structure is symmetric owing to the merging of the asymmetric distribution of points. The significant and quite similar contributions from Cl⋯H/H⋯Cl contacts to the Hirshfeld surfaces of A, B and overall (I)
, Fig. 9
(d), have very little influence on the molecular packing due to their interatomic distances being equal to or greater than the sum of their van der Waals radii. The linear distribution of points beginning from de + di ∼3.3 and 3.4 Å, Fig. 9
(e), in the Cl⋯Cl delineated plots for A and B, respectively, indicate the presence of Cl⋯Cl interactions. The small contribution from C⋯C contacts to the Hirshfeld surface of (I)
has a negligible effect on the packing.
Comparable fingerprint plots for (II)
are shown in Fig. 10
and percentage contributions are collected in Table 4
. The short interatomic H⋯H contact between symmetry-related ethylene-H8B atoms is viewed as a single peak at de + di ∼2.2 Å in Fig. 10
(b). In Fig. 10
(c), delineated into C⋯H/H⋯C contacts, Table 3
, the forceps-like tips at de + di ∼2.6 Å reflect the significant C—H⋯π contacts in the molecular packing. The contribution of Br⋯H/H⋯Br contacts to the Hirshfeld surface of (II)
, Fig. 10
(d), have very little influence on the packing due to their interatomic distances being around the sum of their van der Waals radii. The short interatomic Br⋯Br contacts in (II)
are viewed as a thin, linear distribution of points initiating from de + di ∼3.5 Å, Fig. 10
(e). As for (I)
, the small contribution from C⋯C contacts to the Hirshfeld surface of (II)
has a negligible effect in the crystal.
| Figure 10 (a) The full two-dimensional fingerprint plot for (II) , and (b)–(e) those delineated into H⋯H, C⋯H/H⋯C, Br⋯Br and Br⋯H/H⋯Br contacts. |
5. Computational chemistry
The pairwise interaction energies between the molecules in the crystals of (I)
and (II)
were calculated by summing up four energy components, being electrostatic (Eele), polarization (Epol), dispersion (Edis) and exchange-repulsion (Erep) (Turner et al., 2017
). These energies were obtained by using the wave functions calculated at the B3LYP/6-31G(d,p) level theory for (I)
and the HF/STO-3G level theory for (II)
. The individual energy components as well as total interaction energy relative to reference molecule within molecular clusters out to 3.8 Å. The nature and strength of the energies for the key identified intermolecular interactions are quantitatively summarized in Table 5
. Dispersive components are dominant as conventional hydrogen bonding is not possible.
Contact | Eele | Epol | Edis | Erep | Etot | (I) | | | | | | Cl1A⋯Cl1A | −0.9 | 0.0 | −3.3 | 7.6 | 0.9 | Cl1B⋯Cl1B | −0.9 | −0.1 | −3.4 | 5.8 | −0.4 | C5—H5⋯Cg(C1A–C6A) | −8.5 | −1.5 | −33.5 | 26.1 | −23.1 | C5⋯H2B | −3.7 | −0.8 | −18.2 | 12.9 | −12.3 | (II) | | | | | | Br1⋯Br2 | −2.2 | −0.1 | −4.9 | 8.4 | 0.1 | C3—H3⋯Cg(C9–C14) | −14.6 | −4.7 | −62.4 | 38.3 | −43.2 | C6—H6⋯Cg(C1–C6) | −5.6 | −1.5 | −25.1 | 15.5 | −16.7 | C13—H13⋯Cg(C9–C14) | −8.9 | −1.9 | −30.9 | 14.2 | −25.9 | H11⋯C6 | −5.0 | −3.2 | −50.6 | 24.7 | −32.7 | H8B⋯H8B | −5.0 | −3.2 | −50.6 | 24.7 | −32.7 | | |
The significant contributions from the C—H⋯π interaction and short interatomic C⋯H/H⋯C contacts in the crystal of (I)
are evident from Table 5
. Also notable, are the negligible energies associated with the Cl⋯Cl contacts due to the dominance of repulsive contributions. With respect to (II)
, it is evident from the comparison of the dispersive component as well as total energies for the different interactions that the strength of interactions in the crystal depend upon distance between the respective molecules. The short Br⋯Br contacts in (II)
also have very small interaction energies.
The magnitudes of intermolecular energies are represented graphically in the energy frameworks of Fig. 11
. Here, the supramolecular architecture of each crystal is viewed through the cylinders joining the centroids of molecular pairs. The red (Eele), green (Edisp) and blue (Etot) colour scheme represent the specified energy components. The radii of the cylinders are proportional to the magnitude of interaction energies which are adjusted with a cut-off value of 2 kJ mol−1 within 4 × 4 × 4 unit cells. The energy frameworks constructed for the clusters about the independent molecules A and B of (I)
as well as that for (II)
also indicate the distinct mode of supramolecular association around the molecules in the molecular packing. The small effect of the electrostatic components and the significant influence of the dispersive components are clearly evident from the energy frameworks shown in Fig. 11
.
| Figure 11 A comparison of the energy frameworks composed of (a) electrostatic potential force, (b) dispersion force and (c) total energy for cluster about a reference molecule of A and B of (I) , and for (II) . The energy frameworks were adjusted to the same scale factor of 80 with a cut-off value of 2 kJ mol−1 within 4 × 4 × 4 unit cells. |
6. Database survey
There are only four halo-substituted 1,2-bis(phenyl)ethylene derivatives in the literature. The key structural parameters for these are summarized in Table 6
. Only one literature structure is not disposed about a centre of inversion, namely the non-symmetric, mixed-halo structure (4-Br,2,6-F2C6H2)CH2CH2C6H4Br-4 (Galán et al., 2016
). Generally, the central Ce—Ce (e = ethylene) bonds are long in these compounds with the exception being the pentabromo derivative, C6Br5CH2CH2C6Br5 (Köppen et al., 2007
).
Ring 1 | Ring 2 | Symmetry | CH2—CH2 | dihedral angle C6/C6 | Reference | 2-BrC6H4 | 2-BrC6H4 | ![[\overline{1}]](teximages/hb7814fi5.gif) | 1.540 (7) | 0 | Kahr et al. (1995 ) | C6F5 | C6F5 | ![[\overline{1}]](teximages/hb7814fi5.gif) | 1.542 (3) | 0 | Krafczyk et al. (1997 ) | C6Br5 | C6Br5 | ![[\overline{1}]](teximages/hb7814fi5.gif) | 1.495 (13) | 0 | Köppen et al. (2007 ) | 4-Br,2,6-F2C6H2 | 4-BrC6H4 | – | 1.522 (10) | 1.67 (16) | Galán et al. (2016 ) | 4-ClC6H4a | 4-ClC6H4 | ![[\overline{1}]](teximages/hb7814fi5.gif) | 1.530 (2) | 0 | This work | | | ![[\overline{1}]](teximages/hb7814fi5.gif) | 1.530 (3) | 0 | | 4-BrC6H4 | 4-BrC6H4 | – | 1.516 (3) | 59.29 (11) | This work | Notes: (a) Two independent molecules comprise the asymmetric unit. | |
8. Refinement
Crystal data, data collection and structure refinement details are summarized in Table 7
. The carbon-bound H atoms were placed in calculated positions (C—H = 0.93–0.99 Å) and were included in the refinement in the riding-model approximation, with Uiso(H) set to 1.2Ueq(C). In the refinement of (II)
, owing to poor agreement the (111) reflection was omitted from the final cycles of refinement.
| (I) | (II) | Crystal data | Chemical formula | C14H12Cl2 | C14H12Br2 | Mr | 251.14 | 340.06 | Crystal system, space group | Monoclinic, C2/c | Monoclinic, P21/c | Temperature (K) | 296 | 100 | a, b, c (Å) | 26.6755 (19), 9.3259 (7), 10.0405 (8) | 10.8761 (2), 7.5157 (1), 15.6131 (3) | β (°) | 99.560 (4) | 106.177 (1) | V (Å3) | 2463.1 (3) | 1225.71 (4) | Z | 8 | 4 | Radiation type | Mo Kα | Mo Kα | μ (mm−1) | 0.50 | 6.58 | Crystal size (mm) | 0.30 × 0.20 × 0.10 | 0.20 × 0.11 × 0.07 | | Data collection | Diffractometer | Bruker SMART APEX CCD area detector | Bruker SMART APEX CCD area detector | Absorption correction | Multi-scan (SADABS; Sheldrick, 1996 ) | Multi-scan (SADABS; Sheldrick, 1996 ) | Tmin, Tmax | 0.623, 0.746 | 0.546, 0.746 | No. of measured, independent and observed [I > 2σ(I)] reflections | 12061, 3090, 2627 | 11908, 3065, 2520 | Rint | 0.026 | 0.035 | (sin θ/λ)max (Å−1) | 0.669 | 0.669 | | Refinement | R[F2 > 2σ(F2)], wR(F2), S | 0.033, 0.090, 1.04 | 0.026, 0.058, 1.03 | No. of reflections | 3090 | 3065 | No. of parameters | 145 | 145 | H-atom treatment | H-atom parameters constrained | H-atom parameters constrained | Δρmax, Δρmin (e Å−3) | 0.31, −0.27 | 0.45, −0.41 | Computer programs: APEX2 (Bruker, 2008 ), SAINT (Bruker, 2008 ), SHELXS97 (Sheldrick, 2008 ), SHELXL2018/3 (Sheldrick, 2015 ), ORTEP-3 for Windows (Farrugia, 2012 ), DIAMOND (Brandenburg, 2006 ), QMol (Gans & Shalloway, 2001 ) and publCIF (Westrip, 2010 ). | |
Supporting information
For both structures, data collection: APEX2 (Bruker, 2008); cell refinement: SAINT (Bruker, 2008); data reduction: SAINT (Bruker, 2008); program(s) used to solve structure: SHELXS97 (Sheldrick, 2008); program(s) used to refine structure: SHELXL2018/3 (Sheldrick, 2015); molecular graphics: ORTEP-3 for Windows (Farrugia, 2012), DIAMOND (Brandenburg, 2006) and QMol (Gans & Shalloway, 2001); software used to prepare material for publication: publCIF (Westrip, 2010).
1-Chloro-4-[2-(4-chlorophenyl)ethyl]benzene (I)
top Crystal data top C14H12Cl2 | F(000) = 1040 |
Mr = 251.14 | Dx = 1.354 Mg m−3 |
Monoclinic, C2/c | Mo Kα radiation, λ = 0.71073 Å |
a = 26.6755 (19) Å | Cell parameters from 4470 reflections |
b = 9.3259 (7) Å | θ = 3.1–28.3° |
c = 10.0405 (8) Å | µ = 0.50 mm−1 |
β = 99.560 (4)° | T = 296 K |
V = 2463.1 (3) Å3 | Prism, colourless |
Z = 8 | 0.30 × 0.20 × 0.10 mm |
Data collection top Bruker model? CCD area detector diffractometer | 3090 independent reflections |
Radiation source: fine-focus sealed tube | 2627 reflections with I > 2σ(I) |
Graphite monochromator | Rint = 0.026 |
φ and ω scans | θmax = 28.4°, θmin = 1.6° |
Absorption correction: multi-scan (SADABS; Sheldrick, 1996) | h = −35→34 |
Tmin = 0.623, Tmax = 0.746 | k = −11→12 |
12061 measured reflections | l = −13→13 |
Refinement top Refinement on F2 | Primary atom site location: structure-invariant direct methods |
Least-squares matrix: full | Hydrogen site location: inferred from neighbouring sites |
R[F2 > 2σ(F2)] = 0.033 | H-atom parameters constrained |
wR(F2) = 0.090 | w = 1/[σ2(Fo2) + (0.046P)2 + 1.4907P] where P = (Fo2 + 2Fc2)/3 |
S = 1.04 | (Δ/σ)max = 0.001 |
3090 reflections | Δρmax = 0.31 e Å−3 |
145 parameters | Δρmin = −0.27 e Å−3 |
0 restraints | |
Special details top Geometry. All esds (except the esd in the dihedral angle between two l.s. planes) are estimated using the full covariance matrix. The cell esds are taken into account individually in the estimation of esds in distances, angles and torsion angles; correlations between esds in cell parameters are only used when they are defined by crystal symmetry. An approximate (isotropic) treatment of cell esds is used for estimating esds involving l.s. planes. |
Fractional atomic coordinates and isotropic or equivalent isotropic displacement parameters (Å2) top | x | y | z | Uiso*/Ueq | |
Cl1A | 0.30558 (2) | 0.69571 (4) | 0.08421 (3) | 0.02990 (11) | |
C1A | 0.35616 (5) | 0.64904 (15) | 0.20984 (13) | 0.0213 (3) | |
C2A | 0.39856 (5) | 0.73741 (15) | 0.23346 (13) | 0.0227 (3) | |
H2A | 0.400392 | 0.819329 | 0.181667 | 0.027* | |
C3A | 0.43825 (5) | 0.70121 (14) | 0.33594 (13) | 0.0224 (3) | |
H3A | 0.466935 | 0.759394 | 0.351988 | 0.027* | |
C4A | 0.43591 (5) | 0.57952 (14) | 0.41510 (13) | 0.0202 (3) | |
C5A | 0.39306 (5) | 0.49193 (14) | 0.38683 (13) | 0.0224 (3) | |
H5A | 0.391223 | 0.409246 | 0.437578 | 0.027* | |
C6A | 0.35314 (5) | 0.52569 (15) | 0.28454 (13) | 0.0237 (3) | |
H6A | 0.324808 | 0.466334 | 0.266505 | 0.028* | |
C7A | 0.47884 (5) | 0.54105 (15) | 0.52636 (13) | 0.0236 (3) | |
H7A1 | 0.465754 | 0.482765 | 0.592893 | 0.028* | |
H7A2 | 0.492801 | 0.628077 | 0.570743 | 0.028* | |
Cl1B | 0.44971 (2) | 0.90465 (4) | 0.91449 (4) | 0.03360 (12) | |
C1B | 0.39561 (5) | 0.87925 (14) | 0.79317 (14) | 0.0229 (3) | |
C2B | 0.34836 (6) | 0.90808 (15) | 0.82606 (14) | 0.0271 (3) | |
H2B | 0.345284 | 0.938736 | 0.912478 | 0.033* | |
C3B | 0.30571 (5) | 0.89060 (15) | 0.72836 (15) | 0.0274 (3) | |
H3B | 0.273843 | 0.910620 | 0.749858 | 0.033* | |
C4B | 0.30929 (5) | 0.84387 (14) | 0.59888 (13) | 0.0223 (3) | |
C5B | 0.35742 (5) | 0.81356 (15) | 0.56992 (14) | 0.0254 (3) | |
H5B | 0.360595 | 0.780451 | 0.484341 | 0.031* | |
C6B | 0.40064 (5) | 0.83161 (15) | 0.66564 (14) | 0.0258 (3) | |
H6B | 0.432599 | 0.811998 | 0.644554 | 0.031* | |
C7B | 0.26239 (5) | 0.82320 (15) | 0.49401 (15) | 0.0272 (3) | |
H7B1 | 0.271471 | 0.832325 | 0.404772 | 0.033* | |
H7B2 | 0.238029 | 0.898025 | 0.504017 | 0.033* | |
Atomic displacement parameters (Å2) top | U11 | U22 | U33 | U12 | U13 | U23 |
Cl1A | 0.02150 (17) | 0.0400 (2) | 0.02582 (18) | 0.00466 (13) | −0.00301 (12) | 0.00464 (14) |
C1A | 0.0173 (6) | 0.0266 (7) | 0.0191 (6) | 0.0037 (5) | 0.0005 (5) | −0.0009 (5) |
C2A | 0.0226 (6) | 0.0237 (6) | 0.0222 (6) | 0.0005 (5) | 0.0051 (5) | 0.0011 (5) |
C3A | 0.0186 (6) | 0.0257 (6) | 0.0230 (6) | −0.0014 (5) | 0.0041 (5) | −0.0029 (5) |
C4A | 0.0175 (6) | 0.0246 (6) | 0.0186 (6) | 0.0046 (5) | 0.0031 (5) | −0.0031 (5) |
C5A | 0.0230 (6) | 0.0206 (6) | 0.0236 (6) | 0.0016 (5) | 0.0035 (5) | 0.0009 (5) |
C6A | 0.0199 (6) | 0.0247 (7) | 0.0258 (7) | −0.0028 (5) | 0.0017 (5) | −0.0024 (5) |
C7A | 0.0199 (6) | 0.0292 (7) | 0.0207 (6) | 0.0045 (5) | 0.0003 (5) | −0.0019 (5) |
Cl1B | 0.02872 (19) | 0.0322 (2) | 0.0356 (2) | −0.00182 (13) | −0.00707 (15) | −0.00290 (14) |
C1B | 0.0223 (6) | 0.0199 (6) | 0.0251 (6) | −0.0016 (5) | 0.0001 (5) | 0.0008 (5) |
C2B | 0.0296 (7) | 0.0296 (7) | 0.0233 (7) | −0.0023 (5) | 0.0076 (6) | −0.0057 (5) |
C3B | 0.0214 (6) | 0.0308 (7) | 0.0313 (7) | −0.0006 (5) | 0.0084 (5) | −0.0031 (6) |
C4B | 0.0211 (6) | 0.0203 (6) | 0.0250 (6) | −0.0005 (5) | 0.0024 (5) | 0.0028 (5) |
C5B | 0.0279 (7) | 0.0278 (7) | 0.0215 (6) | 0.0031 (5) | 0.0067 (5) | −0.0011 (5) |
C6B | 0.0216 (6) | 0.0280 (7) | 0.0286 (7) | 0.0041 (5) | 0.0069 (5) | 0.0002 (5) |
C7B | 0.0251 (7) | 0.0269 (7) | 0.0276 (7) | −0.0002 (5) | −0.0011 (6) | 0.0033 (6) |
Geometric parameters (Å, º) top Cl1A—C1A | 1.7424 (13) | Cl1B—C1B | 1.7432 (13) |
C1A—C6A | 1.3829 (19) | C1B—C2B | 1.381 (2) |
C1A—C2A | 1.3877 (18) | C1B—C6B | 1.383 (2) |
C2A—C3A | 1.3899 (18) | C2B—C3B | 1.383 (2) |
C2A—H2A | 0.9300 | C2B—H2B | 0.9300 |
C3A—C4A | 1.3928 (19) | C3B—C4B | 1.3895 (19) |
C3A—H3A | 0.9300 | C3B—H3B | 0.9300 |
C4A—C5A | 1.3952 (18) | C4B—C5B | 1.3917 (18) |
C4A—C7A | 1.5043 (17) | C4B—C7B | 1.5079 (18) |
C5A—C6A | 1.3873 (18) | C5B—C6B | 1.3832 (19) |
C5A—H5A | 0.9300 | C5B—H5B | 0.9300 |
C6A—H6A | 0.9300 | C6B—H6B | 0.9300 |
C7A—C7Ai | 1.530 (2) | C7B—C7Bii | 1.530 (3) |
C7A—H7A1 | 0.9700 | C7B—H7B1 | 0.9700 |
C7A—H7A2 | 0.9700 | C7B—H7B2 | 0.9700 |
| | | |
C6A—C1A—C2A | 121.37 (12) | C2B—C1B—C6B | 121.11 (12) |
C6A—C1A—Cl1A | 119.51 (10) | C2B—C1B—Cl1B | 119.26 (11) |
C2A—C1A—Cl1A | 119.12 (10) | C6B—C1B—Cl1B | 119.63 (10) |
C1A—C2A—C3A | 118.77 (12) | C3B—C2B—C1B | 118.90 (13) |
C1A—C2A—H2A | 120.6 | C3B—C2B—H2B | 120.6 |
C3A—C2A—H2A | 120.6 | C1B—C2B—H2B | 120.6 |
C2A—C3A—C4A | 121.30 (12) | C2B—C3B—C4B | 121.62 (13) |
C2A—C3A—H3A | 119.4 | C2B—C3B—H3B | 119.2 |
C4A—C3A—H3A | 119.4 | C4B—C3B—H3B | 119.2 |
C3A—C4A—C5A | 118.28 (12) | C3B—C4B—C5B | 117.94 (12) |
C3A—C4A—C7A | 121.15 (12) | C3B—C4B—C7B | 121.02 (12) |
C5A—C4A—C7A | 120.55 (12) | C5B—C4B—C7B | 121.03 (12) |
C6A—C5A—C4A | 121.33 (12) | C6B—C5B—C4B | 121.43 (13) |
C6A—C5A—H5A | 119.3 | C6B—C5B—H5B | 119.3 |
C4A—C5A—H5A | 119.3 | C4B—C5B—H5B | 119.3 |
C5A—C6A—C1A | 118.92 (12) | C5B—C6B—C1B | 118.99 (12) |
C5A—C6A—H6A | 120.5 | C5B—C6B—H6B | 120.5 |
C1A—C6A—H6A | 120.5 | C1B—C6B—H6B | 120.5 |
C4A—C7A—C7Ai | 112.14 (13) | C4B—C7B—C7Bii | 112.23 (14) |
C4A—C7A—H7A1 | 109.2 | C4B—C7B—H7B1 | 109.2 |
C7Ai—C7A—H7A1 | 109.2 | C7Bii—C7B—H7B1 | 109.2 |
C4A—C7A—H7A2 | 109.2 | C4B—C7B—H7B2 | 109.2 |
C7Ai—C7A—H7A2 | 109.2 | C7Bii—C7B—H7B2 | 109.2 |
H7A1—C7A—H7A2 | 107.9 | H7B1—C7B—H7B2 | 107.9 |
| | | |
C6A—C1A—C2A—C3A | −0.98 (19) | C6B—C1B—C2B—C3B | −1.0 (2) |
Cl1A—C1A—C2A—C3A | 178.52 (10) | Cl1B—C1B—C2B—C3B | 178.62 (11) |
C1A—C2A—C3A—C4A | −0.5 (2) | C1B—C2B—C3B—C4B | 0.5 (2) |
C2A—C3A—C4A—C5A | 1.63 (19) | C2B—C3B—C4B—C5B | 0.5 (2) |
C2A—C3A—C4A—C7A | −179.71 (12) | C2B—C3B—C4B—C7B | 179.08 (13) |
C3A—C4A—C5A—C6A | −1.28 (19) | C3B—C4B—C5B—C6B | −1.2 (2) |
C7A—C4A—C5A—C6A | −179.95 (12) | C7B—C4B—C5B—C6B | −179.72 (13) |
C4A—C5A—C6A—C1A | −0.2 (2) | C4B—C5B—C6B—C1B | 0.8 (2) |
C2A—C1A—C6A—C5A | 1.3 (2) | C2B—C1B—C6B—C5B | 0.3 (2) |
Cl1A—C1A—C6A—C5A | −178.18 (10) | Cl1B—C1B—C6B—C5B | −179.24 (11) |
C3A—C4A—C7A—C7Ai | −83.46 (19) | C3B—C4B—C7B—C7Bii | −83.7 (2) |
C5A—C4A—C7A—C7Ai | 95.17 (17) | C5B—C4B—C7B—C7Bii | 94.75 (19) |
Symmetry codes: (i) −x+1, −y+1, −z+1; (ii) −x+1/2, −y+3/2, −z+1. |
Hydrogen-bond geometry (Å, º) topCg1 is the centroid of the (C1A–C6A) ring. |
D—H···A | D—H | H···A | D···A | D—H···A |
C5B—H5B···Cg1 | 0.93 | 2.62 | 3.4866 (15) | 155 |
1-Bromo-4-[2-(4-chlorophenyl)ethyl]benzene (II)
top Crystal data top C14H12Br2 | F(000) = 664 |
Mr = 340.06 | Dx = 1.843 Mg m−3 |
Monoclinic, P21/c | Mo Kα radiation, λ = 0.71073 Å |
a = 10.8761 (2) Å | Cell parameters from 3449 reflections |
b = 7.5157 (1) Å | θ = 2.9–28.3° |
c = 15.6131 (3) Å | µ = 6.58 mm−1 |
β = 106.177 (1)° | T = 100 K |
V = 1225.71 (4) Å3 | Prism, colourless |
Z = 4 | 0.20 × 0.11 × 0.07 mm |
Data collection top Bruker model? CCD area detector diffractometer | 3065 independent reflections |
Radiation source: fine-focus sealed tube | 2520 reflections with I > 2σ(I) |
Graphite monochromator | Rint = 0.035 |
φ and ω scans | θmax = 28.4°, θmin = 2.7° |
Absorption correction: multi-scan (SADABS; Sheldrick, 1996) | h = −14→14 |
Tmin = 0.546, Tmax = 0.746 | k = −10→9 |
11908 measured reflections | l = −20→20 |
Refinement top Refinement on F2 | Primary atom site location: structure-invariant direct methods |
Least-squares matrix: full | Hydrogen site location: inferred from neighbouring sites |
R[F2 > 2σ(F2)] = 0.026 | H-atom parameters constrained |
wR(F2) = 0.058 | w = 1/[σ2(Fo2) + (0.0289P)2 + 0.0779P] where P = (Fo2 + 2Fc2)/3 |
S = 1.03 | (Δ/σ)max = 0.001 |
3065 reflections | Δρmax = 0.45 e Å−3 |
145 parameters | Δρmin = −0.40 e Å−3 |
0 restraints | |
Special details top Geometry. All esds (except the esd in the dihedral angle between two l.s. planes) are estimated using the full covariance matrix. The cell esds are taken into account individually in the estimation of esds in distances, angles and torsion angles; correlations between esds in cell parameters are only used when they are defined by crystal symmetry. An approximate (isotropic) treatment of cell esds is used for estimating esds involving l.s. planes. |
Refinement. Owing to poor agreement, the (1 1 1) reflection was omitted from the final cycles of refinement. |
Fractional atomic coordinates and isotropic or equivalent isotropic displacement parameters (Å2) top | x | y | z | Uiso*/Ueq | |
Br1 | 0.50841 (2) | 0.84764 (3) | 0.90903 (2) | 0.02370 (8) | |
Br2 | −0.25048 (2) | 0.70383 (3) | 0.09773 (2) | 0.02253 (8) | |
C1 | 0.4150 (2) | 0.8055 (3) | 0.78827 (15) | 0.0159 (5) | |
C2 | 0.3013 (2) | 0.7135 (3) | 0.77050 (15) | 0.0161 (4) | |
H2 | 0.269796 | 0.672242 | 0.817887 | 0.019* | |
C3 | 0.2335 (2) | 0.6817 (3) | 0.68274 (15) | 0.0177 (5) | |
H3 | 0.155194 | 0.617644 | 0.670292 | 0.021* | |
C4 | 0.2778 (2) | 0.7417 (3) | 0.61232 (15) | 0.0167 (5) | |
C5 | 0.3933 (2) | 0.8356 (3) | 0.63289 (16) | 0.0187 (5) | |
H5 | 0.425069 | 0.878319 | 0.585874 | 0.022* | |
C6 | 0.4624 (2) | 0.8674 (3) | 0.72041 (15) | 0.0173 (5) | |
H6 | 0.541066 | 0.930801 | 0.733576 | 0.021* | |
C7 | 0.2051 (2) | 0.7034 (4) | 0.51691 (16) | 0.0255 (6) | |
H7A | 0.218424 | 0.577129 | 0.503799 | 0.031* | |
H7B | 0.241041 | 0.777365 | 0.477256 | 0.031* | |
C8 | 0.0625 (2) | 0.7386 (3) | 0.49531 (15) | 0.0212 (5) | |
H8A | 0.025232 | 0.652822 | 0.529264 | 0.025* | |
H8B | 0.049949 | 0.859257 | 0.516862 | 0.025* | |
C9 | −0.0116 (2) | 0.7260 (3) | 0.39829 (14) | 0.0147 (4) | |
C10 | −0.1344 (2) | 0.7992 (3) | 0.36922 (15) | 0.0167 (5) | |
H10 | −0.170004 | 0.854105 | 0.411688 | 0.020* | |
C11 | −0.2056 (2) | 0.7945 (3) | 0.28122 (15) | 0.0172 (5) | |
H11 | −0.288380 | 0.846752 | 0.263034 | 0.021* | |
C12 | −0.1540 (2) | 0.7119 (3) | 0.21965 (15) | 0.0161 (4) | |
C13 | −0.0336 (2) | 0.6354 (3) | 0.24522 (15) | 0.0172 (5) | |
H13 | 0.000460 | 0.578442 | 0.202509 | 0.021* | |
C14 | 0.0369 (2) | 0.6430 (3) | 0.33433 (15) | 0.0154 (4) | |
H14 | 0.119669 | 0.590830 | 0.352166 | 0.018* | |
Atomic displacement parameters (Å2) top | U11 | U22 | U33 | U12 | U13 | U23 |
Br1 | 0.02109 (13) | 0.02960 (15) | 0.01712 (13) | −0.00317 (9) | −0.00014 (9) | −0.00484 (9) |
Br2 | 0.01971 (13) | 0.03068 (15) | 0.01471 (12) | −0.00164 (9) | 0.00065 (9) | 0.00265 (9) |
C1 | 0.0149 (11) | 0.0157 (12) | 0.0151 (11) | 0.0040 (8) | 0.0009 (9) | −0.0027 (8) |
C2 | 0.0160 (11) | 0.0163 (11) | 0.0175 (11) | 0.0019 (8) | 0.0073 (9) | 0.0018 (9) |
C3 | 0.0136 (11) | 0.0205 (12) | 0.0185 (12) | −0.0004 (8) | 0.0036 (9) | −0.0009 (9) |
C4 | 0.0146 (11) | 0.0200 (12) | 0.0152 (11) | 0.0023 (8) | 0.0036 (9) | −0.0006 (9) |
C5 | 0.0146 (11) | 0.0216 (12) | 0.0214 (12) | 0.0015 (9) | 0.0076 (9) | 0.0038 (9) |
C6 | 0.0106 (10) | 0.0172 (12) | 0.0243 (12) | −0.0004 (8) | 0.0053 (9) | −0.0012 (9) |
C7 | 0.0160 (12) | 0.0436 (16) | 0.0171 (12) | 0.0027 (10) | 0.0050 (10) | −0.0026 (11) |
C8 | 0.0166 (12) | 0.0281 (13) | 0.0172 (12) | 0.0018 (10) | 0.0021 (9) | −0.0023 (10) |
C9 | 0.0169 (11) | 0.0132 (11) | 0.0146 (11) | −0.0018 (8) | 0.0054 (9) | 0.0007 (8) |
C10 | 0.0173 (11) | 0.0152 (12) | 0.0195 (12) | −0.0002 (8) | 0.0082 (9) | 0.0003 (9) |
C11 | 0.0129 (10) | 0.0172 (12) | 0.0211 (12) | 0.0006 (8) | 0.0039 (9) | 0.0026 (9) |
C12 | 0.0160 (11) | 0.0175 (12) | 0.0133 (10) | −0.0038 (8) | 0.0015 (9) | 0.0025 (9) |
C13 | 0.0189 (11) | 0.0165 (12) | 0.0174 (11) | −0.0011 (9) | 0.0070 (9) | −0.0003 (9) |
C14 | 0.0129 (11) | 0.0160 (11) | 0.0176 (11) | −0.0012 (8) | 0.0048 (9) | 0.0015 (9) |
Geometric parameters (Å, º) top Br1—C1 | 1.902 (2) | C7—H7B | 0.9900 |
Br2—C12 | 1.901 (2) | C8—C9 | 1.507 (3) |
C1—C2 | 1.376 (3) | C8—H8A | 0.9900 |
C1—C6 | 1.382 (3) | C8—H8B | 0.9900 |
C2—C3 | 1.384 (3) | C9—C10 | 1.399 (3) |
C2—H2 | 0.9500 | C9—C14 | 1.399 (3) |
C3—C4 | 1.393 (3) | C10—C11 | 1.377 (3) |
C3—H3 | 0.9500 | C10—H10 | 0.9500 |
C4—C5 | 1.398 (3) | C11—C12 | 1.388 (3) |
C4—C7 | 1.507 (3) | C11—H11 | 0.9500 |
C5—C6 | 1.385 (3) | C12—C13 | 1.384 (3) |
C5—H5 | 0.9500 | C13—C14 | 1.390 (3) |
C6—H6 | 0.9500 | C13—H13 | 0.9500 |
C7—C8 | 1.516 (3) | C14—H14 | 0.9500 |
C7—H7A | 0.9900 | | |
| | | |
C2—C1—C6 | 121.4 (2) | C9—C8—C7 | 116.1 (2) |
C2—C1—Br1 | 119.02 (17) | C9—C8—H8A | 108.3 |
C6—C1—Br1 | 119.57 (17) | C7—C8—H8A | 108.3 |
C1—C2—C3 | 119.2 (2) | C9—C8—H8B | 108.3 |
C1—C2—H2 | 120.4 | C7—C8—H8B | 108.3 |
C3—C2—H2 | 120.4 | H8A—C8—H8B | 107.4 |
C2—C3—C4 | 121.3 (2) | C10—C9—C14 | 117.4 (2) |
C2—C3—H3 | 119.4 | C10—C9—C8 | 119.73 (19) |
C4—C3—H3 | 119.4 | C14—C9—C8 | 122.9 (2) |
C3—C4—C5 | 117.9 (2) | C11—C10—C9 | 122.3 (2) |
C3—C4—C7 | 121.1 (2) | C11—C10—H10 | 118.8 |
C5—C4—C7 | 120.9 (2) | C9—C10—H10 | 118.8 |
C6—C5—C4 | 121.3 (2) | C10—C11—C12 | 118.6 (2) |
C6—C5—H5 | 119.3 | C10—C11—H11 | 120.7 |
C4—C5—H5 | 119.3 | C12—C11—H11 | 120.7 |
C1—C6—C5 | 118.8 (2) | C13—C12—C11 | 121.3 (2) |
C1—C6—H6 | 120.6 | C13—C12—Br2 | 119.32 (17) |
C5—C6—H6 | 120.6 | C11—C12—Br2 | 119.41 (17) |
C4—C7—C8 | 114.2 (2) | C12—C13—C14 | 119.0 (2) |
C4—C7—H7A | 108.7 | C12—C13—H13 | 120.5 |
C8—C7—H7A | 108.7 | C14—C13—H13 | 120.5 |
C4—C7—H7B | 108.7 | C13—C14—C9 | 121.4 (2) |
C8—C7—H7B | 108.7 | C13—C14—H14 | 119.3 |
H7A—C7—H7B | 107.6 | C9—C14—H14 | 119.3 |
| | | |
C6—C1—C2—C3 | 0.3 (3) | C7—C8—C9—C10 | −163.7 (2) |
Br1—C1—C2—C3 | −179.64 (16) | C7—C8—C9—C14 | 16.4 (3) |
C1—C2—C3—C4 | −0.3 (3) | C14—C9—C10—C11 | −1.3 (3) |
C2—C3—C4—C5 | 0.0 (3) | C8—C9—C10—C11 | 178.8 (2) |
C2—C3—C4—C7 | 178.7 (2) | C9—C10—C11—C12 | 0.9 (3) |
C3—C4—C5—C6 | 0.4 (3) | C10—C11—C12—C13 | 0.0 (3) |
C7—C4—C5—C6 | −178.3 (2) | C10—C11—C12—Br2 | −179.99 (16) |
C2—C1—C6—C5 | 0.0 (3) | C11—C12—C13—C14 | −0.5 (3) |
Br1—C1—C6—C5 | 179.96 (16) | Br2—C12—C13—C14 | 179.49 (16) |
C4—C5—C6—C1 | −0.4 (3) | C12—C13—C14—C9 | 0.1 (3) |
C3—C4—C7—C8 | 46.6 (3) | C10—C9—C14—C13 | 0.7 (3) |
C5—C4—C7—C8 | −134.8 (2) | C8—C9—C14—C13 | −179.4 (2) |
C4—C7—C8—C9 | 172.1 (2) | | |
Hydrogen-bond geometry (Å, º) topCg1 and Cg2 are the centroids of the (C1–C6) and (C9–C14) rings, respectively. |
D—H···A | D—H | H···A | D···A | D—H···A |
C3—H3···Cg2i | 0.95 | 2.69 | 3.442 (2) | 136 |
C6—H6···Cg1ii | 0.95 | 2.91 | 3.704 (2) | 141 |
C13—H13···Cg2iii | 0.95 | 2.87 | 3.569 (2) | 131 |
Symmetry codes: (i) −x, −y+1, −z+1; (ii) −x+1, y+1/2, −z+3/2; (iii) −x, y−1/2, −z+1/2. |
Summary of short interatomic contacts (Å) in (I)a topContact | Distance | Symmetry operation |
(I) | | |
H6B···H72A | 2.35 | x, y, z |
H5B···C2A | 2.75 | x, y, z |
H5B···C3A | 2.72 | x, y, z |
H2B···C5B | 2.67 | x, 2 - y, 1/2 + z |
C11A···Cl1A | 3.3184 (7) | 1/2 - x, 3/2 - y, -z |
Cl1B···Cl1B | 3.4322 (7) | 1 - x, 2 - y, 2 - z |
(II) | | |
H8B···H8B | 2.21 | -x, 2 - y, 1 - z |
H3···C13 | 2.74 | -x, 1 - y, 1 - z |
H3···C14 | 2.72 | -x, 1 - y, 1 - z |
H6···C1 | 2.82 | 1 - x, 1/2 + y, 3/2 - z |
H6···C2 | 2.62 | 1 - x, 1/2 + y, 3/2 - z |
H11···C6 | 2.80 | -x, 2 - y, 1 - z |
Br1···Br2 | 3.5242 (4) | 1 + x, y, 1 + z |
Notes: (a) The interatomic distances are calculated in Crystal Explorer (Turner et al., 2017) whereby the X—H bond lengths are adjusted to their neutron values. |
Percentage contributions of interatomic contacts to the Hirshfeld surface for (I) and (II) topContact | Percentage contribution | | | |
| (I) - molecule A | (I) - molecule B | (I) | (II) |
H···H | 30.8 | 35.1 | 31.4 | 30.6 |
C···H/H···C | 32.5 | 27.0 | 28.4 | 32.7 |
X···H/H···X | 30.5 | 33.3 | 34.2 | 30.4 |
X···X | 3.9 | 2.2 | 3.4 | 4.9 |
C···C | 1.3 | 1.3 | 1.4 | 0.0 |
C···X/X···C | 1.1 | 1.1 | 1.2 | 1.4 |
Summary of interaction energies (kJ mol-1) calculated for (I) and (II) topContact | Eele | Epol | Edis | Erep | Etot |
(I) | | | | | |
Cl1A···Cl1A | -0.9 | 0.0 | -3.3 | 7.6 | 0.9 |
Cl1B···Cl1B | -0.9 | -0.1 | -3.4 | 5.8 | -0.4 |
C5—H5···Cg(C1A–C6A) | -8.5 | -1.5 | -33.5 | 26.1 | -23.1 |
C5···H2B | -3.7 | -0.8 | -18.2 | 12.9 | -12.3 |
(II) | | | | | |
Br1···Br2 | -2.2 | -0.1 | -4.9 | 8.4 | 0.1 |
C3—H3···Cg(C9–C14) | -14.6 | -4.7 | -62.4 | 38.3 | -43.2 |
C6—H6···Cg(C1–C6) | -5.6 | -1.5 | -25.1 | 15.5 | -16.7 |
C13—H13···Cg(C9–C14) | -8.9 | -1.9 | -30.9 | 14.2 | -25.9 |
H11···C6 | -5.0 | -3.2 | -50.6 | 24.7 | -32.7 |
H8B···H8B | -5.0 | -3.2 | -50.6 | 24.7 | -32.7 |
Geometric data (Å, °) for halo-substituted 1,2-bis(phenyl)ethane structures topRing 1 | Ring 2 | Symmetry | CH2—CH2 | dihedral angle C6/C6 | Reference |
2-BrC6H4 | 2-BrC6H4 | 1 | 1.540 (7) | 0 | Kahr et al. (1995) |
C6F5 | C6F5 | 1 | 1.542 (3) | 0 | Krafczyk et al. (1997) |
C6Br5 | C6Br5 | 1 | 1.495 (13) | 0 | Köppen et al. (2007) |
4-Br,2,6-F2C6H2 | 4-BrC6H4 | – | 1.522 (10) | 1.67 (16) | Galán et al. (2016) |
4-ClC6H4a | 4-ClC6H4 | 1 | 1.530 (2) | 0 | This work |
| | 1 | 1.530 (3) | 0 | |
4-BrC6H4 | 4-BrC6H4 | – | 1.516 (3) | 59.29 (11) | This work |
Notes: (a) Two independent molecules comprise the asymmetric unit. |
Acknowledgements
Sunway University Sdn Bhd is thanked for support.
References
Bestiuc, I., Buruiana, T., Idriceanu, S., Popescu, V. & Caraculacu, A. (1985). Rev. Chim. 36, 621–623. CAS Google Scholar
Brandenburg, K. (2006). DIAMOND. Crystal Impact GbR, Bonn, Germany. Google Scholar
Bruker (2008). APEX2 and SAINT. Bruker AXS Inc., Madison, Wisconsin, USA. Google Scholar
Farrugia, L. J. (2012). J. Appl. Cryst. 45, 849–854. Web of Science CrossRef CAS IUCr Journals Google Scholar
Galán, E., Perrin, M. L., Lutz, M., van der Zant, H. S. J., Grozema, F. C. & Eelkema, R. (2016). Org. Biomol. Chem. 14, 2439–2443. PubMed Google Scholar
Gans, J. & Shalloway, D. (2001). J. Mol. Graphics Modell. 19, 557–559. Web of Science CrossRef CAS Google Scholar
Golden, J. H. (1961). J. Chem. Soc. pp. 1604–1610. CrossRef Google Scholar
Hu, Y.-L., Li, F., Gu, G.-L. & Lu, M. (2011). Catal. Lett. 141, 467–473. CrossRef CAS Google Scholar
Kahr, B., Mitchell, C. A., Chance, J. M., Clark, R. V., Gantzel, P., Baldridge, K. K. & Siegel, J. S. (1995). J. Am. Chem. Soc. 117, 4479–4482. CSD CrossRef CAS Web of Science Google Scholar
Köppen, R., Emmerling, F. & Becker, R. (2007). Acta Cryst. E63, o585–o586. Web of Science CSD CrossRef IUCr Journals Google Scholar
Krafczyk, R., Thönnessen, H., Jones, P. G. & Schmutzler, R. (1997). J. Fluor. Chem. 83, 159–166. CSD CrossRef CAS Google Scholar
Liu, J. & Li, B. (2007). Synth. Commun. 37, 3273–3278. CrossRef CAS Google Scholar
Otsubo, T., Ogura, F., Yamaguchi, H., Higuchi, H. & Misumi, S. (1980). Synth. Commun. 10, 595–601. CrossRef CAS Google Scholar
Parnes, Z. N., Romanova, V. S. & Vol'pin, M. E. (1989). Zh. Org. Khim. 25, 1075–1079. CAS Google Scholar
Remizov, A. B., Kamalova, D. I. & Stolov, A. A. (2005). Russ. J. Phys. Chem. A, 79(Suppl. 1), 76–80. Google Scholar
Sheldrick, G. M. (1996). SADABS. University of Göttingen, Germany. Google Scholar
Sheldrick, G. M. (2008). Acta Cryst. A64, 112–122. Web of Science CrossRef CAS IUCr Journals Google Scholar
Sheldrick, G. M. (2015). Acta Cryst. C71, 3–8. Web of Science CrossRef IUCr Journals Google Scholar
Sisido, K., Takeda, Y. & Kinugawa, Z. (1961). J. Am. Chem. Soc. 83, 538–541. CrossRef Web of Science Google Scholar
Tan, S. L., Jotani, M. M. & Tiekink, E. R. T. (2019). Acta Cryst. E75, 308–318. Web of Science CrossRef IUCr Journals Google Scholar
Turner, M. J., Mckinnon, J. J., Wolff, S. K., Grimwood, D. J., Spackman, P. R., Jayatilaka, D. & Spackman, M. A. (2017). Crystal Explorer 17. The University of Western Australia. Google Scholar
Westrip, S. P. (2010). J. Appl. Cryst. 43, 920–925. Web of Science CrossRef CAS IUCr Journals Google Scholar
This is an open-access article distributed under the terms of the Creative Commons Attribution (CC-BY) Licence, which permits unrestricted use, distribution, and reproduction in any medium, provided the original authors and source are cited.
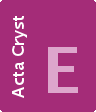 | CRYSTALLOGRAPHIC COMMUNICATIONS |
ISSN: 2056-9890
Open

access